Investigating sex differences in genetic relatedness in great-tailed grackles in Tempe, Arizona to infer potential sex biases in dispersal
Sevchik, A., Logan, C. J., McCune, K. B., Blackwell, A., Rowney, C. and Lukas, D
https://doi.org/10.32942/osf.io/t6beh
Dispersal: from “neutral” to a state- and context-dependent view
Recommended by Emanuel A. Fronhofer based on reviews by 2 anonymous reviewers
Traditionally, dispersal has often been seen as “random” or “neutral” as Lowe & McPeek (2014) have put it. This simplistic view is likely due to dispersal being intrinsically difficult to measure empirically as well as “random” dispersal being a convenient simplifying assumption in theoretical work. Clobert et al. (2009), and many others, have highlighted how misleading this assumption is. Rather, dispersal seems to be usually a complex reaction norm, depending both on internal as well as external factors. One such internal factor is the sex of the dispersing individual. A recent review of the theoretical literature (Li & Kokko 2019) shows that while ideas explaining sex-biased dispersal go back over 40 years this state-dependency of dispersal is far from comprehensively understood.
Sevchik et al. (2021) tackle this challenge empirically in a bird species, the great-tailed grackle. In contrast to most bird species, where females disperse more than males, the authors report genetic evidence indicating male-biased dispersal. The authors argue that this difference can be explained by the great-tailed grackle’s social and mating-system.
Dispersal is a central life-history trait (Bonte & Dahirel 2017) with major consequences for ecological and evolutionary processes and patterns. Therefore, studies like Sevchik et al. (2021) are valuable contributions for advancing our understanding of spatial ecology and evolution. Importantly, Sevchik et al. also lead to way to a more open and reproducible science of ecology and evolution. The authors are among the pioneers of preregistering research in their field and their way of doing research should serve as a model for others.
References
Bonte, D. & Dahirel, M. (2017) Dispersal: a central and independent trait in life history. Oikos 126: 472-479. doi: https://doi.org/10.1111/oik.03801
Clobert, J., Le Galliard, J. F., Cote, J., Meylan, S. & Massot, M. (2009) Informed dispersal, heterogeneity in animal dispersal syndromes and the dynamics of spatially structured populations. Ecol. Lett.: 12, 197-209. doi: https://doi.org/10.1111/j.1461-0248.2008.01267.x
Li, X.-Y. & Kokko, H. (2019) Sex-biased dispersal: a review of the theory. Biol. Rev. 94: 721-736. doi: https://doi.org/10.1111/brv.12475
Lowe, W. H. & McPeek, M. A. (2014) Is dispersal neutral? Trends Ecol. Evol. 29: 444-450. doi: https://doi.org/10.1016/j.tree.2014.05.009
Sevchik, A., Logan, C. J., McCune, K. B., Blackwell, A., Rowney, C. & Lukas, D. (2021) Investigating sex differences in genetic relatedness in great-tailed grackles in Tempe, Arizona to infer potential sex biases in dispersal. EcoEvoRxiv, osf.io/t6beh, ver. 5 peer-reviewed and recommended by Peer community in Ecology. doi: https://doi.org/10.32942/osf.io/t6beh
| Investigating sex differences in genetic relatedness in great-tailed grackles in Tempe, Arizona to infer potential sex biases in dispersal | Sevchik, A., Logan, C. J., McCune, K. B., Blackwell, A., Rowney, C. and Lukas, D | <p>In most bird species, females disperse prior to their first breeding attempt, while males remain closer to the place they hatched for their entire lives. Explanations for such female bias in natal dispersal have focused on the resource-defense ... | 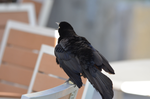 | Behaviour & Ethology, Dispersal & Migration, Zoology | Emanuel A. Fronhofer | | 2020-08-24 17:53:06 | View |
“Hidden” natural selection and the evolution of body size in harvested stocks
Recommended by Simon Blanchet based on reviews by Jean-François Arnoldi and 1 anonymous reviewer
Humans are exploiting biological resources since thousands of years. Exploitation of biological resources has become particularly intense since the beginning of the 20th century and the steep increase in the worldwide human population size. Marine and freshwater fishes are not exception to that rule, and they have been (and continue to be) strongly harvested as a source of proteins for humans. For some species, fishery has been so intense that natural stocks have virtually collapsed in only a few decades. The worst example begin that of the Northwest Atlantic cod that has declined by more than 95% of its historical biomasses in only 20-30 years of intensive exploitation (Frank et al. 2005). These rapid and steep changes in biomasses have huge impacts on the entire ecosystems since species targeted by fisheries are often at the top of trophic chains (Frank et al. 2005).
Beyond demographic impacts, fisheries also have evolutionary impacts on populations, which can also indirectly alter ecosystems (Uusi-Heikkilä et al. 2015; Palkovacs et al. 2018). Fishermen generally focus on the largest specimens, and hence exert a strong selective pressure against these largest fish (which is called “harvest selection”). There is now ample evidence that harvest selection can lead to rapid evolutionary changes in natural populations toward small individuals (Kuparinen & Festa-Bianchet 2017). These evolutionary changes are of course undesirable from a human perspective, and have attracted many scientific questions. Nonetheless, the consequence of harvest selection is not always observable in natural populations, and there are cases in which no phenotypic change (or on the contrary an increase in mean body size) has been observed after intense harvest pressures. In a conceptual Essay, Edeline and Loeuille (Edeline & Loeuille 2020) propose novel ideas to explain why the evolutionary consequences of harvest selection can be so diverse, and how a cross talk between ecological and evolutionary dynamics can explain patterns observed in natural stocks.
The general and novel concept proposed by Edeline and Loeuille is actually as old as Darwin’s book; The Origin of Species (Darwin 1859). It is based on the simple idea that natural selection acting on harvested populations can actually be strong, and counter-balance (or on the contrary reinforce) the evolutionary consequence of harvest selection. Although simple, the idea that natural and harvest selection are jointly shaping contemporary evolution of exploited populations lead to various and sometimes complex scenarios that can (i) explain unresolved empirical patterns and (ii) refine predictions regarding the long-term viability of exploited populations.
The Edeline and Loeuille’s crafty inspiration is that natural selection acting on exploited populations is itself an indirect consequence of harvest (Edeline & Loeuille 2020). They suggest that, by modifying the size structure of populations (a key parameter for ecological interactions), harvest indirectly alters interactions between populations and their biotic environment through competition and predation, which changes the ecological theatre and hence the selective pressures acting back to populations. They named this process “size-dependent eco-evolutionary feedback loops” and develop several scenarios in which these feedback loops ultimately deviate the evolutionary outcome of harvest selection from expectation. The scenarios they explore are based on strong theoretical knowledge, and range from simple ones in which a single species (the harvest species) is evolving to more complex (and realistic) ones in which multiple (e.g. the harvest species and its prey) species are co-evolving.
I will not come into the details of each scenario here, and I will let the readers (re-)discovering the complex beauty of biological life and natural selection. Nonetheless, I will emphasize the importance of considering these eco-evolutionary processes altogether to fully grasp the response of exploited populations. Edeline and Loeuille convincingly demonstrate that reduced body size due to harvest selection is obviously not the only response of exploited fish populations when natural selection is jointly considered (Edeline & Loeuille 2020). On the contrary, they show that –under some realistic ecological circumstances relaxing exploitative competition due to reduced population densities- natural selection can act antagonistically, and hence favour stable body size in exploited populations. Although this seems further desirable from a human perspective than a downsizing of exploited populations, it is actually mere window dressing as Edeline and Loeuille further showed that this response is accompanied by an erosion of the evolvability –and hence a lowest probability of long-term persistence- of these exploited populations.
Humans, by exploiting biological resources, are breaking the relative equilibrium of complex entities, and the response of populations to this disturbance is itself often complex and heterogeneous. In this Essay, Edeline and Loeuille provide –under simple terms- the theoretical and conceptual bases required to improve predictions regarding the evolutionary responses of natural populations to exploitation by humans (Edeline & Loeuille 2020). An important next step will be to generate data and methods allowing confronting the empirical reality to these novel concepts (e.g. (Monk et al. 2021), so as to identify the most likely evolutionary scenarios sustaining biological responses of exploited populations, and hence to set the best management plans for the long-term sustainability of these populations.
References
Darwin, C. (1859). On the Origin of Species by Means of Natural Selection. John Murray, London.
Edeline, E. & Loeuille, N. (2021) Size-dependent eco-evolutionary feedbacks in fisheries. bioRxiv, 2020.04.03.022905, ver. 4 peer-reviewed and recommended by PCI Ecology. doi: https://doi.org/10.1101/2020.04.03.022905
Frank, K.T., Petrie, B., Choi, J. S. & Leggett, W.C. (2005). Trophic Cascades in a Formerly Cod-Dominated Ecosystem. Science, 308, 1621–1623. doi: https://doi.org/10.1126/science.1113075
Kuparinen, A. & Festa-Bianchet, M. (2017). Harvest-induced evolution: insights from aquatic and terrestrial systems. Philos. Trans. R. Soc. B Biol. Sci., 372, 20160036. doi: https://doi.org/10.1098/rstb.2016.0036
Monk, C.T., Bekkevold, D., Klefoth, T., Pagel, T., Palmer, M. & Arlinghaus, R. (2021). The battle between harvest and natural selection creates small and shy fish. Proc. Natl. Acad. Sci., 118, e2009451118. doi: https://doi.org/10.1073/pnas.2009451118
Palkovacs, E.P., Moritsch, M.M., Contolini, G.M. & Pelletier, F. (2018). Ecology of harvest-driven trait changes and implications for ecosystem management. Front. Ecol. Environ., 16, 20–28. doi: https://doi.org/10.1002/fee.1743
Uusi-Heikkilä, S., Whiteley, A.R., Kuparinen, A., Matsumura, S., Venturelli, P.A., Wolter, C., et al. (2015). The evolutionary legacy of size-selective harvesting extends from genes to populations. Evol. Appl., 8, 597–620. doi: https://doi.org/10.1111/eva.12268
| Size-dependent eco-evolutionary feedbacks in fisheries | Eric Edeline and Nicolas Loeuille | <p>Harvesting may drive body downsizing along with population declines and decreased harvesting yields. These changes are commonly construed as direct consequences of harvest selection, where small-bodied, early-reproducing individuals are immedia... | 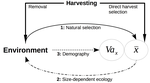 | Biodiversity, Community ecology, Competition, Eco-evolutionary dynamics, Evolutionary ecology, Food webs, Interaction networks, Life history, Population ecology, Theoretical ecology | Simon Blanchet | | 2020-04-03 16:14:05 | View |
Consistent variations in personality traits and their potential for genetic improvement of biocontrol agents: Trichogramma evanescens as a case study
Silène Lartigue, Myriam Yalaoui, Jean Belliard, Claire Caravel, Louise Jeandroz, Géraldine Groussier, Vincent Calcagno, Philippe Louâpre, François-Xavier Dechaume-Moncharmont, Thibaut Malausa and Jérôme Moreau
https://doi.org/10.1101/2020.08.21.257881
Tell us how you can be, and we’ll make you better: exploiting genetic variability in personality traits to improve top-down control of agricultural pests
Recommended by Marta Montserrat based on reviews by Bart A Pannebakker, François Dumont, Joshua Patrick Byrne and Ana Pimenta Goncalves Pereira
Agriculture in the XXI century faces the huge challenge of having to provide food to a rapidly growing human population, which is expected to reach 10.9 billion in 2100 (UUNN 2019), by means of practices and methods that guarantee crop sustainability, human health safety, and respect to the environment (UUNN 2015). Such regulation by the United Nations ultimately entails that agricultural scientists are urged to design strategies and methods that effectively minimize the use of harmful chemical products to control pest populations and to improve soil quality.
One of the most, if not the most, sustainable, safe, and environmentally friendly approach to apply against pests is Biological Pest Control (BPC, hereafter), that is, the use of natural enemies to control the populations of pest organisms. The concept of BPC is by no means new: long back to the 300 AC, Chinese farmers built bamboo bridges between citrus trees to facilitate the foraging of the ant species Oecophylla smaragdina to control lepidopteran citrus pests (Konishi and Ito, 1973); It is also nice to use this recommendation letter to recall and quote the words written in 1752 by the famous Swedish taxonomist, botanist and zoologist, Carl Linnaeus: "Every insect has its predator which follows and destroys it. Such predatory insects should be caught and used for disinfecting crop-plants" (Hörstadius (1974) apud Linnaeus 1752).
Acknowledging the many cases of successes from BPC along our recent history, it is also true that application of BPC strategies during the XX century suffered from wrong-doings, mainly when the introduced biological control agent (BCA, hereafter) was of exotic origin and with a generalist diet-breath; in some cases the release of exotic species resulted on global extinction, reduction in the range of distribution, reduction in the population abundance, and partial displacement, of native and functionally similar species, and interbreeding with them (reviewed in van Lenteren et al. 2006). One of the most famous cases is that of Harmonia axyridis, a coccinellid predator of Asian origin that caused important environmental damage in North America (reviewed in Koch & Galvan, 2008).
Fortunately, after the implementation of the Nagoya protocol (CBD, 2011) importation of exotic species for BPC use was severely restricted and controlled, worldwide. Consequently, companies and agricultural scientist were driven to reinforce their focus and interest on the exploitation of native natural enemies, via the mass-rearing and release of native candidates (augmentative BPC), the conservation of landscapes near the crops to provide resources for natural enemies (i.e. conservation biological pest control), or via the exploitation of the genetic variability of BCAs, to create strains performing better at regulating pest populations under specific biotic or abiotic negative circumstances. Some of these cases are cited in Lartigue et al. (2020). The genetic improvement of BCAs is a strategy still in its infancy, but there is no doubt that the interest for it has significantly increased over the last 5 years (Lommen et al 2017, Bielza 2020, Leung et al 2020).
In my humble opinion, what makes the paper of Lartigue et al. (2020) a remarkable contribution to the field of genetic breeding of BCAs is that it opens a new window of opportunities to the field, by exploring the possibilities for artificial selection of behavioral traits (Réale et al. 2007) to "create" strains of natural enemies displaying behavioral syndromes (Sih et al. 2004) that makes them better at regulating pest populations. The behavioral approach for breeding BCAs can then be extended by crossing it with known abiotic and/or biotic hostile environments (e.g. warm and drought environments, presence of predators/competitors to the BCA, respectively) and engineer strains more prompt to display particular behavioral syndromes to help them to overcome the overall hostility of specific environments. I strongly believe that the approach proposed in Lartigue et al. (2020) will influence the future management of agricultural systems, where strategies including the genetic breeding of BCAs’ behavior will contribute to create better guards and protectors of our crops.
References
Bielza, P., Balanza, V., Cifuentes, D. and Mendoza, J. E. (2020). Challenges facing arthropod biological control: Identifying traits for genetic improvement of predators in protected crops. Pest Manag Sci. doi: https://doi.org/10.1002/ps.5857
CBD - Convention on Biological Diversity, 2011. The Nagoya Protocol on Access and Benefit-sharing, https://www.cbd.int/abs/doc/protocol/nagoya-protocol-en.pdf
Hörstadius, S. (1974). Linnaeus, animals and man. Biological Journal of the Linnaean Society, 6, 269-275. doi: https://doi.org/10.1111/j.1095-8312.1974.tb00725.x
Koch, R.L. and Galvan, T.L. (2008). Bad side of a good beetle: the North American experience with Harmonia axyridis. BioControl 53, 23–35. doi: https://doi.org/10.1007/978-1-4020-6939-0_3
Konishi, M. and Ito, Y. (1973). Early entomology in East Asia. In: Smith, R.F., Mittler, T.E., Smith, C.N. (Eds.), History of Entomology, Annual Reviews Inc., Palo Alto, California, pp. 1-20.
Lartigue, S., Yalaoui, M., Belliard, J., Caravel, C., Jeandroz, L., Groussier, G., Calcagno, V., Louâpre, P., Dechaume-Moncharmont, F.-X., Malausa, T. and Moreau, J. (2020). Consistent variations in personality traits and their potential for genetic improvement of biocontrol agents: Trichogramma evanescens as a case study. bioRxiv, 2020.08.21.257881, ver. 4 peer-reviewed and recommended by PCI Ecology. doi: https://doi.org/10.1101/2020.08.21.257881
Leung et al. (2020). Next-generation biological control: the need for integrating genetics and genomics. Biological Reviews, 95(6), 1838–1854. doi: https://doi.org/10.1111/brv.12641
Lommen, S. T. E., de Jong, P. W. and Pannebakker, B. A. (2017). It is time to bridge the gap between exploring and exploiting: prospects for utilizing intraspecific genetic variation to optimize arthropods for augmentative pest control – a review. Entomologia Experimentalis et Applicata, 162: 108-123. doi: https://doi.org/10.1111/eea.12510
Réale, D., Reader, S. M., Sol, D., McDougall, P. T. and Dingemanse, N. J. (2007). Integrating animal temperament within ecology and evolution. Biological Reviews, 82: 291-318. doi: https://doi.org/10.1111/j.1469-185X.2007.00010.x
Sih, A., Bell, A. and Johnson, J. C. (2004). Behavioral syndromes: an ecological and evolutionary overview. Trends in Ecology and Evolution, 19(7), 372–378. doi: https://doi.org/10.1016/j.tree.2004.04.009
UUNN. 2015. Transforming our world: the 2030 Agenda for Sustainable Development. report of the Open Working Group of the General Assembly on Sustainable Development Goals (A/68/970 and Corr.1; see also A/68/970/Add.1–3).
UUNN. 2019. World population prospects 2019. United Nations, Department of Economic and Social Affairs, Population Division: Highlights. ST/ESA/SER.A/423.
van Lenteren, J. C., Bale, J., Bigler, F., Hokkanen, H. M. T. and Loomans A. J. M. (2006). Assessing risks of releasing exotic biological control agents of arthropod pests. Annual Review of Entomology, 51: 609-634. doi: https://doi.org/10.1146/annurev.ento.51.110104.151129
| Consistent variations in personality traits and their potential for genetic improvement of biocontrol agents: Trichogramma evanescens as a case study | Silène Lartigue, Myriam Yalaoui, Jean Belliard, Claire Caravel, Louise Jeandroz, Géraldine Groussier, Vincent Calcagno, Philippe Louâpre, François-Xavier Dechaume-Moncharmont, Thibaut Malausa and Jérôme Moreau | <p>Improvements in the biological control of agricultural pests require improvements in the phenotyping methods used by practitioners to select efficient biological control agent (BCA) populations in industrial rearing or field conditions. Consist... | 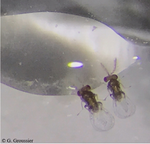 | Agroecology, Behaviour & Ethology, Biological control, Evolutionary ecology, Life history | Marta Montserrat | | 2020-08-24 10:40:03 | View |
Comparing statistical and mechanistic models to identify the drivers of mortality within a rear-edge beech population
Cathleen Petit-Cailleux, Hendrik Davi, François Lefevre, Christophe Hurson, Joseph Garrigue, Jean-André Magdalou, Elodie Magnanou and Sylvie Oddou-Muratorio
https://doi.org/10.1101/645747
The complexity of predicting mortality in trees
Recommended by Lucía DeSoto based on reviews by Lisa Hülsmann and 2 anonymous reviewers
One of the main issues of forest ecosystems is rising tree mortality as a result of extreme weather events (Franklin et al., 1987). Eventually, tree mortality reduces forest biomass (Allen et al., 2010), although its effect on forest ecosystem fluxes seems not lasting too long (Anderegg et al., 2016). This controversy about the negative consequences of tree mortality is joined to the debate about the drivers triggering and the mechanisms accelerating tree decline. For instance, there is still room for discussion about carbon starvation or hydraulic failure determining the decay processes (Sevanto et al., 2014) or about the importance of mortality sources (Reichstein et al., 2013). Therefore, understanding and predicting tree mortality has become one of the challenges for forest ecologists in the last decade, doubling the rate of articles published on the topic (*). Although predicting the responses of ecosystems to environmental change based on the traits of species may seem a simplistic conception of ecosystem functioning (Sutherland et al., 2013), identifying those traits that are involved in the proneness of a tree to die would help to predict how forests will respond to climate threatens.
Modelling tree mortality is complex, involving multiple factors acting simultaneously at different scales, from tree genetics to ecosystem dynamics and from microsite conditions to global climatic events. Therefore, taking into account different approaches to reduce uncertainty of the predictions is needed (Bugmann et al., 2019). Petit-Cailleux et al. (2020) uses statistical and process-based models to detect the main mortality drivers of a drought- and frost-prone beech population. Particularly, they assessed the intra-individual characteristics of the population, that may play a decisive role explaining the differences in tree vulnerability to extreme weather events. Comparing the results of both analytical approaches, they find out several key factors, such as defoliation, leaf phenology and tree size, that were consistent between them. Even more, the process-based model showed the physiological mechanisms that may explain the individual vulnerability, for instance higher loss of hydraulic conductance may increase the mortality risk of trees with early budburst phenology and large stem diameter. The authors also successfully model annual mortality rate with a linear relationship including only three parameters: loss of conductance, biomass of reserves and late frost days.
This valuable study is a good example of the complexity in understanding and predicting tree mortality. The authors carried out the ambitious commitment of studying the inter-annual variation in mortality with 14-year dataset. However, it might be not enough time to control for the dependence of temporal data to soundly model mortality rate. The authors also acknowledge that the use of two approaches increases the knowledge from different perspectives, but at the same time comparing their results is difficult because the parameters used are not identical. Particularly, process-based models tend to consider the same microclimatic conditions for every tree in the population, and may produce inconsistences with statistical models. Alternatively, individual-based modelling might overcome some of the incompatibilities between the approaches (Zhu et al., 2019).
(*) Number (and percentage) of articles found in Web of Sciences after searching (December the 10th, 2020) “tree mortality”: from 163 (0.006%) in 2010 to 412 (0.013%) in 2020.
References
Allen et al. (2010). A global overview of drought and heat-induced tree mortality reveals emerging climate change risks for forests. Forest ecology and management, 259(4), 660-684. doi: https://doi.org/10.1016/j.foreco.2009.09.001
Anderegg et al. (2016). When a tree dies in the forest: scaling climate-driven tree mortality to ecosystem water and carbon fluxes. Ecosystems, 19(6), 1133-1147. doi: https://doi.org/10.1007/s10021-016-9982-1
Bugmann et al. (2019). Tree mortality submodels drive simulated long‐term forest dynamics: assessing 15 models from the stand to global scale. Ecosphere, 10(2), e02616. doi: https://doi.org/10.1002/ecs2.2616
Franklin, J. F., Shugart, H. H. and Harmon, M. E. (1987) Death as an ecological process: the causes, consequences, and variability of tree mortality. BioScience, 37, 550–556. doi: https://doi.org/10.2307/1310665
Petit-Cailleux, C., Davi, H., Lefèvre, F., Garrigue, J., Magdalou, J.-A., Hurson, C., Magnanou, E. and Oddou-Muratorio, S. (2020) Comparing statistical and mechanistic models to identify the drivers of mortality within a rear-edge beech population. bioRxiv, 645747, ver 7 peer-reviewed and recommended by Peer Community in Ecology. https://doi.org/10.1101/645747
Reichstein et al. (2013). Climate extremes and the carbon cycle. Nature, 500(7462), 287-295. doi: https://doi.org/10.1038/nature12350
Sevanto, S., Mcdowell, N. G., Dickman, L. T., Pangle, R., and Pockman, W. T. (2014). How do trees die? A test of the hydraulic failure and carbon starvation hypotheses. Plant, cell & environment, 37(1), 153-161. doi: https://doi.org/10.1111/pce.12141
Sutherland et al. (2013). Identification of 100 fundamental ecological questions. Journal of ecology, 101(1), 58-67. doi: https://doi.org/10.1111/1365-2745.12025
Zhu, Y., Liu, Z., and Jin, G. (2019). Evaluating individual-based tree mortality modeling with temporal observation data collected from a large forest plot. Forest Ecology and Management, 450, 117496. doi: https://doi.org/10.1016/j.foreco.2019.117496
| Comparing statistical and mechanistic models to identify the drivers of mortality within a rear-edge beech population | Cathleen Petit-Cailleux, Hendrik Davi, François Lefevre, Christophe Hurson, Joseph Garrigue, Jean-André Magdalou, Elodie Magnanou and Sylvie Oddou-Muratorio | <p>Since several studies have been reporting an increase in the decline of forests, a major issue in ecology is to better understand and predict tree mortality. The interactions between the different factors and the physiological processes giving ... | 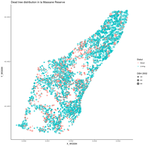 | Climate change, Physiology, Population ecology | Lucía DeSoto | | 2019-05-24 11:37:38 | View |
Assessing bat-vehicle collision risks using acoustic 3D tracking
Recommended by Gloriana Chaverri based on reviews by Mark Brigham and ?
The loss of biodiversity is an issue of great concern, especially if the extinction of species or the loss of a large number of individuals within populations results in a loss of critical ecosystem services. We know that the most important threat to most species is habitat loss and degradation (Keil et al., 2015; Pimm et al., 2014); the latter can be caused by multiple anthropogenic activities, including pollution, introduction of invasive species and fragmentation (Brook et al., 2008; Scanes, 2018). Roads are a major cause of habitat fragmentation, isolating previously connected populations and being a direct source of mortality for animals that attempt to cross them (Spellberg, 1998).
While most studies have focused on the effect of roads on larger mammals (Bartonička et al., 2018; Litvaitis and Tash, 2008), in recent years many researchers have grown increasingly concerned about the risk of collision between bats and vehicles (Fensome and Mathews, 2016). For example, a recent publication by Medinas et al. (2021) found 509 bat casualties along a 51-km-long transect during a period of 3 years. Their study provides extremely valuable information to asses which factors primarily drive bat mortality on roads, yet it required a substantial investment of time coupled with the difficulty of detecting bat carcasses. Other studies have used acoustic monitoring as a proxy to gauge risk of collision based on estimates of bat density along roads (reviewed in Fensome and Mathews 2016); while the results of such studies are valuable, the number of passes recorded does not necessarily equal collision risk, as many species may simply avoid crossing the roads. Understanding the risk of collisions is of vital importance for adequate planning of road construction, particularly for key sites that harbor threatened bat species or unusually large populations, especially if these are already greatly impacted by other anthropogenic activities (e.g. wind turbines; Kunz et al. 2007) or unusually deadly pathogens (e.g. white-nose syndrome; Blehert et al. 2009).
The study by Roemer et al. (2020) titled “Influence of local landscape and time of year on bat-road collision risks”, is a welcome addition to our understanding of bat collision risk as it employs a more accurate assessment of bat collision risk based on acoustic monitoring and tracking of flight paths. The goal of the study of Roemer and collaborators, which was conducted at 66 study sites in the Mediterranean region, is to provide an assessment of collision risk based on bat activity near roads. They collected a substantial amount of information for several species: more than 30,000 estimated flight trajectories for 21+ species, including Barbastella barbastellus, Myotis spp., Plecotus sp., Rhinolophus ferrumequinum, Miniopterus schreibersii, Pipistrellus spp., Nyctalus leisleri, and others. They assess risk based on estimates of 1) species abundance from acoustic monitoring, 2) direction of flight paths along roads, and 3) bat-vehicle co-occurrence.
Their findings suggest that risk is habitat, species, guild, and season-specific. Roads within forested habitats posed the largest threats for most species, particularly since most flights within these habitats occurred at the zone of collision risk. They also found that bats typically fly parallel to the road axis regardless of habitat type, which they argue supports the idea that bats may use roads as corridors. The results of their study, as expected, also show that the majority of bat passes were detected during summer or autumn, depending on species, yet they provide novel findings of an increase in risky behaviors during autumn, when the number of passes at the zone of collision risk increased significantly. Their results also suggest that mid-range echolocators, a classification that is based on call design and parameters (Frey-Ehrenbold et al., 2013), had a larger portion of flights in the zone at risk, thus potentially making them more susceptible than short and long-range echolocators to collisions with vehicles.
The methods employed by Roemer et al. (2020) could further help us determine how roads pose species and site-specific threats in a diversity of places without the need to invest a significant amount of time locating bat carcasses. Their findings are also important as they could provide valuable information for deciding where new roads should be constructed, particularly if the most vulnerable species are abundant, perhaps due to the presence of important roost sites. They also show how habitats near larger roads could increase threats, providing an important first step for recommendations regarding road construction and maintenance. As pointed out by one reviewer, one possible limitation of the study is that the results are not supported by the identification of carcasses. For example, does an increase in the number of identified flights at the zone of risk really translate into an increase in the number of collisions? Regardless of the latter, the paper’s methods and results are very valuable and provide an important step towards developing additional tools to assess bat-vehicle collision risks.
References
[1] Bartonička T, Andrášik R, Duľa M, Sedoník J, Bíl M (2018) Identification of local factors causing clustering of animal-vehicle collisions. The Journal of Wildlife Management, 82, 940–947. https://doi.org/10.1002/jwmg.21467
[2] Blehert DS, Hicks AC, Behr M, Meteyer CU, Berlowski-Zier BM, Buckles EL, Coleman JTH, Darling SR, Gargas A, Niver R, Okoniewski JC, Rudd RJ, Stone WB (2009) Bat White-Nose Syndrome: An Emerging Fungal Pathogen? Science, 323, 227–227. https://doi.org/10.1126/science.1163874
[3] Brook BW, Sodhi NS, Bradshaw CJA (2008) Synergies among extinction drivers under global change. Trends in Ecology & Evolution, 23, 453–460. https://doi.org/10.1016/j.tree.2008.03.011
[4] Fensome AG, Mathews F (2016) Roads and bats: a meta-analysis and review of the evidence on vehicle collisions and barrier effects. Mammal Review, 46, 311–323. https://doi.org/10.1111/mam.12072
[5] Frey‐Ehrenbold A, Bontadina F, Arlettaz R, Obrist MK (2013) Landscape connectivity, habitat structure and activity of bat guilds in farmland-dominated matrices. Journal of Applied Ecology, 50, 252–261. https://doi.org/10.1111/1365-2664.12034
[6] Keil P, Storch D, Jetz W (2015) On the decline of biodiversity due to area loss. Nature Communications, 6, 8837. https://doi.org/10.1038/ncomms9837
[7] Kunz TH, Arnett EB, Erickson WP, Hoar AR, Johnson GD, Larkin RP, Strickland MD, Thresher RW, Tuttle MD (2007) Ecological impacts of wind energy development on bats: questions, research needs, and hypotheses. Frontiers in Ecology and the Environment, 5, 315–324. https://doi.org/10.1890/1540-9295(2007)5[315:EIOWED]2.0.CO;2
[8] Litvaitis JA, Tash JP (2008) An Approach Toward Understanding Wildlife-Vehicle Collisions. Environmental Management, 42, 688–697. https://doi.org/10.1007/s00267-008-9108-4
[9] Medinas D, Marques JT, Costa P, Santos S, Rebelo H, Barbosa AM, Mira A (2021) Spatiotemporal persistence of bat roadkill hotspots in response to dynamics of habitat suitability and activity patterns. Journal of Environmental Management, 277, 111412. https://doi.org/10.1016/j.jenvman.2020.111412
[10] Pimm SL, Jenkins CN, Abell R, Brooks TM, Gittleman JL, Joppa LN, Raven PH, Roberts CM, Sexton JO (2014) The biodiversity of species and their rates of extinction, distribution, and protection. Science, 344. https://doi.org/10.1126/science.1246752
[11] Roemer C, Coulon A, Disca T, Bas Y (2020) Influence of local landscape and time of year on bat-road collision risks. bioRxiv, 2020.07.15.204115, ver. 3 peer-reviewed and recommended by Peer Community in Ecology. https://doi.org/10.1101/2020.07.15.204115
[12] Scanes CG (2018) Chapter 19 - Human Activity and Habitat Loss: Destruction, Fragmentation, and Degradation. In: Animals and Human Society (eds Scanes CG, Toukhsati SR), pp. 451–482. Academic Press. https://doi.org/10.1016/B978-0-12-805247-1.00026-5
[13] Spellerberg I (1998) Ecological effects of roads and traffic: a literature review. Global Ecology & Biogeography Letters, 7, 317–333. https://doi.org/10.1046/j.1466-822x.1998.00308.x
| Influence of local landscape and time of year on bat-road collision risks | Charlotte Roemer, Aurélie Coulon, Thierry Disca, and Yves Bas | <p>Roads impact bat populations through habitat loss and collisions. High quality habitats particularly increase bat mortalities on roads, yet many questions remain concerning how local landscape features may influence bat behaviour and lead to hi... | 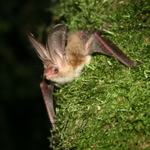 | Behaviour & Ethology, Biodiversity, Conservation biology, Human impact, Landscape ecology | Gloriana Chaverri | | 2020-07-20 10:56:29 | View |
Hough transform implementation to evaluate the morphological variability of the moon jellyfish (Aurelia spp.)
Céline Lacaux, Agnès Desolneux, Justine Gadreaud, Bertrand Martin-Garin and Alain Thiéry
https://doi.org/10.1101/2020.03.11.986984
A new member of the morphometrics jungle to better monitor vulnerable lagoons
Recommended by Vincent Bonhomme based on reviews by Julien Claude and 1 anonymous reviewer
In the recent years, morphometrics, the quantitative description of shape and its covariation [1] gained considerable momentum in evolutionary ecology. Using the form of organisms to describe, classify and try to understand their diversity can be traced back at least to Aristotle. More recently, two successive revolutions rejuvenated this idea [1–3]: first, a proper mathematical refoundation of the theory of shape, then a technical revolution in the apparatus able to acquire raw data. By using a feature extraction method and planning its massive use on data acquired by aerial drones, the study by Lacaux and colleagues [4] retraces this curse of events.
The radial symmetry of Aurelia spp. jelly fish, a common species complex, is affected by stress and more largely by environmental variations, such as pollution exposition. Aurelia spp. normally present four gonads so that the proportion of non-tetramerous individuals in a population has been proposed as a biomarker [5,6].
In this study, the authors implemented the Hough transform to largely automate the detection of the gonads in Aurelia spp. Such use of the Hough transform, a long-used approach to identify shapes through edge detection, is new to morphometrics. Here, the Aurelia spp. gonads are identified as ellipses from which aspect descriptors can be derived, and primarily counted and thus can be used to quantify the proportion of individuals presenting body plans disorders.
The sample sizes studied here were too low to allow finer-grained ecophysiological investigations. That being said, the proof-of-concept is convincing and this paper paths the way for an operational and innovative approach to the ecological monitoring of sensible aquatic ecosystems.
References
[1] Kendall, D. G. (1989). A survey of the statistical theory of shape. Statistical Science, 87-99. doi: https://doi.org/10.1214/ss/1177012589
[2] Rohlf, F. J., and Marcus, L. F. (1993). A revolution morphometrics. Trends in ecology & evolution, 8(4), 129-132. doi: https://doi.org/10.1016/0169-5347(93)90024-J
[3] Adams, D. C., Rohlf, F. J., and Slice, D. E. (2004). Geometric morphometrics: ten years of progress following the ‘revolution’. Italian Journal of Zoology, 71(1), 5-16. doi: https://doi.org/10.1080/11250000409356545
[4] Lacaux, C., Desolneux, A., Gadreaud, J., Martin-Garin, B. and Thiéry, A. (2020) Hough transform implementation to evaluate the morphological variability of the moon jellyfish (Aurelia spp.). bioRxiv, 2020.03.11.986984, ver. 3 peer-reviewed and recommended by Peer Community in Ecology. doi: https://doi.org/10.1101/2020.03.11.986984
[5] Gershwin, L. A. (1999). Clonal and population variation in jellyfish symmetry. Journal of the Marine Biological Association of the United Kingdom, 79(6), 993-1000. doi: https://doi.org/10.1017/S0025315499001228
[6] Gadreaud, J., Martin-Garin, B., Artells, E., Levard, C., Auffan, M., Barkate, A.-L. and Thiéry, A. (2017) The moon jellyfish as a new bioindicator: impact of silver nanoparticles on the morphogenesis. In: Mariottini GL, editor. Jellyfish: ecology, distribution patterns and human interactions. Nova Science Publishers; 2017. pp. 277–292.
| Hough transform implementation to evaluate the morphological variability of the moon jellyfish (Aurelia spp.) | Céline Lacaux, Agnès Desolneux, Justine Gadreaud, Bertrand Martin-Garin and Alain Thiéry | <p>Variations of the animal body plan morphology and morphometry can be used as prognostic tools of their habitat quality. The potential of the moon jellyfish (Aurelia spp.) as a new model organism has been poorly tested. However, as a tetramerous... | 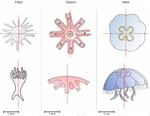 | Morphometrics | Vincent Bonhomme | | 2020-03-18 17:40:51 | View |
Once upon a time in the far south: Influence of local drivers and functional traits on plant invasion in the harsh sub-Antarctic islands
Manuele Bazzichetto, François Massol, Marta Carboni, Jonathan Lenoir, Jonas Johan Lembrechts, Rémi Joly, David Renault
https://doi.org/10.1101/2020.07.19.210880
A meaningful application of species distribution models and functional traits to understand invasion dynamics
Recommended by Joaquín Hortal based on reviews by Paula Matos and Peter Convey
Polar and subpolar regions are fragile environments, where the introduction of alien species may completely change ecosystem dynamics if the alien species become keystone species (e.g. Croll, 2005). The increasing number of human visits, together with climate change, are favouring the introduction and settling of new invaders to these regions, particularly in Antarctica (Hughes et al. 2015). Within this context, the joint use of Species Distribution Models (SDM) –to assess the areas potentially suitable for the aliens– with other measures of the potential to become successful invaders can inform on the need for devoting specific efforts to eradicate these new species before they become naturalized (e.g. Pertierra et al. 2016).
Bazzichetto et al. (2020) use data from a detailed inventory, SDMs and trait data altogether to assess the drivers of invasion success of six alien plants on Possession Island, in the remote sub-Antarctic archipelago of Crozet. SDMs have inherent limitations to describe different aspects of species distributions, including the fundamental niche and, with it, the areas that could host viable populations (Hortal et al. 2012). Therefore, their utility to predict future biological invasions is limited (Jiménez-Valverde et al. 2011). However, they can be powerful tools to describe species range dynamics if they are thoughtfully used by adopting conscious decisions about the techniques and data used, and interpreting carefully the actual implications of their results.
This is what Bazzichetto et al. (2020) do, using General Linear Models (GLM) –a technique well rooted in the original niche-based SDM theory (e.g. Austin 1990)– that can provide a meaningful description of the realized niche within the limits of an adequately sampled region. Further, as alien species share and are similarly affected by several steps of the invasion process (Richardson et al. 2000), these authors model the realized distribution of the six species altogether. This can be done through the recently developed joint-SDM, a group of techniques where the co-occurrence of the modelled species is explicitly taken into account during modelling (e.g. Pollock et al. 2014). Here, the addition of species traits has been identified as a key step to understand the associations of species in space (see Dormann et al. 2018). Bazzichetto et al. (2020) combine their GLM-based SDM for each species with a so-called multi-SDM approach, where they assess together the consistency in the interactions between both species and topographically-driven climate variations, and several plant traits and two key anthropic factors –accessibility from human settlements and distance to hiking paths.
This work is a good example on how a theoretically meaningful SDM approach can provide useful –though perhaps not deep– insights on biological invasions for remote landscapes threatened by biotic homogenization. By combining climate and topographic variables as proxies for the spatial variations in the abiotic conditions regulating plant growth, measures of accessibility, and traits of the plant invaders, Bazzichetto et al. (2020) are able to identify the different effects that the interactions between the potential intensity of propagule dissemination by humans, and the ecological characteristics of the invaders themselves, may have on their invasion success.
The innovation of modelling together species responses is important because it allows dissecting the spatial dynamics of spread of the invaders, which indeed vary according to a handful of their traits. For example, their results show that no all old residents have profited from the larger time of residence in the island, as Poa pratensis is seemingly as dependent of a higher intensity of human activity as the newcomer invaders in general are. According to Bazzichetto et al. trait-based analyses, these differences are apparently related with plant height, as smaller plants disperse more easily. Further, being perennial also provides an advantage for the persistence in areas with less human influence. This puts name, shame and fame to the known influence of plant life history on their dispersal success (Beckman et al. 2018), at least for the particular case of plant invasions in Possession Island.
Of course this approach has limitations, as data on the texture, chemistry and temperature of the soil are not available, and thus were not considered in the analyses. These factors may be critical for both establishment and persistence of small plants in the harsh Antarctic environments, as Bazzichetto et al. (2020) recognize. But all in all, their results provide key insights on which traits may confer alien plants with a higher likelihood of becoming successful invaders in the fragile Antarctic and sub-Antarctic ecosystems. This opens a way for rapid assessments of invasibility, which will help identifying which species in the process of naturalizing may require active contention measures to prevent them from becoming ecological game changers and cause disastrous cascade effects that shift the dynamics of native ecosystems.
References
Austin, M. P., Nicholls, A. O., and Margules, C. R. (1990). Measurement of the realized qualitative niche: environmental niches of five Eucalyptus species. Ecological Monographs, 60(2), 161-177. doi: https://doi.org/10.2307/1943043
Bazzichetto, M., Massol, F., Carboni, M., Lenoir, J., Lembrechts, J. J. and Joly, R. (2020) Once upon a time in the far south: Influence of local drivers and functional traits on plant invasion in the harsh sub-Antarctic islands. bioRxiv, 2020.07.19.210880, ver. 3 peer-reviewed and recommended by PCI Ecology. doi: https://doi.org/10.1101/2020.07.19.210880
Beckman, N. G., Bullock, J. M., and Salguero-Gómez, R. (2018). High dispersal ability is related to fast life-history strategies. Journal of Ecology, 106(4), 1349-1362. doi: https://doi.org/10.1111/1365-2745.12989
Croll, D. A., Maron, J. L., Estes, J. A., Danner, E. M., and Byrd, G. V. (2005). Introduced predators transform subarctic islands from grassland to tundra. Science, 307(5717), 1959-1961. doi: https://doi.org/10.1126/science.1108485
Dormann, C. F., Bobrowski, M., Dehling, D. M., Harris, D. J., Hartig, F., Lischke, H., Moretti, M. D., Pagel, J., Pinkert, S., Schleuning, M., Schmidt, S. I., Sheppard, C. S., Steinbauer, M. J., Zeuss, D., and Kraan, C. (2018). Biotic interactions in species distribution modelling: 10 questions to guide interpretation and avoid false conclusions. Global Ecology and Biogeography, 27(9), 1004-1016. doi: https://doi.org/10.1111/geb.12759
Jiménez-Valverde, A., Peterson, A., Soberón, J., Overton, J., Aragón, P., and Lobo, J. (2011). Use of niche models in invasive species risk assessments. Biological Invasions, 13(12), 2785-2797. doi: https://doi.org/10.1007/s10530-011-9963-4
Hortal, J., Lobo, J. M., and Jiménez-Valverde, A. (2012). Basic questions in biogeography and the (lack of) simplicity of species distributions: Putting species distribution models in the right place. Natureza & Conservação – Brazilian Journal of Nature Conservation, 10(2), 108-118. doi: https://doi.org/10.4322/natcon.2012.029
Hughes, K. A., Pertierra, L. R., Molina-Montenegro, M. A., and Convey, P. (2015). Biological invasions in terrestrial Antarctica: what is the current status and can we respond? Biodiversity and Conservation, 24(5), 1031-1055. doi: https://doi.org/10.1007/s10531-015-0896-6
Pertierra, L. R., Baker, M., Howard, C., Vega, G. C., Olalla-Tarraga, M. A., and Scott, J. (2016). Assessing the invasive risk of two non-native Agrostis species on sub-Antarctic Macquarie Island. Polar Biology, 39(12), 2361-2371. doi: https://doi.org/10.1007/s00300-016-1912-3
Pollock, L. J., Tingley, R., Morris, W. K., Golding, N., O'Hara, R. B., Parris, K. M., Vesk, P. A., and McCarthy, M. A. (2014). Understanding co-occurrence by modelling species simultaneously with a Joint Species Distribution Model (JSDM). Methods in Ecology and Evolution, 5(5), 397-406. doi: https://doi.org/10.1111/2041-210X.12180
Richardson, D. M., Pyšek, P., Rejmánek, M., Barbour, M. G., Panetta, F. D., and West, C. J. (2000). Naturalization and invasion of alien plants: concepts and definitions. Diversity and Distributions, 6(2), 93-107. doi: https://doi.org/10.1046/j.1472-4642.2000.00083.x
| Once upon a time in the far south: Influence of local drivers and functional traits on plant invasion in the harsh sub-Antarctic islands | Manuele Bazzichetto, François Massol, Marta Carboni, Jonathan Lenoir, Jonas Johan Lembrechts, Rémi Joly, David Renault | <p>Aim Here, we aim to: (i) investigate the local effect of environmental and human-related factors on alien plant invasion in sub-Antarctic islands; (ii) explore the relationship between alien species features and their dependence on anthropogeni... | 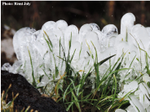 | Biogeography, Biological invasions, Spatial ecology, Metacommunities & Metapopulations, Species distributions | Joaquín Hortal | | 2020-07-21 21:13:08 | View |
Intraspecific diversity loss in a predator species alters prey community structure and ecosystem functions
Allan Raffard, Julien Cucherousset, José M. Montoya, Murielle Richard, Samson Acoca-Pidolle, Camille Poésy, Alexandre Garreau, Frédéric Santoul & Simon Blanchet.
https://doi.org/10.1101/2020.06.10.144337
Hidden diversity: how genetic richness affects species diversity and ecosystem processes in freshwater ponds
Recommended by Frederik De Laender based on reviews by Andrew Barnes and Jes Hines
Biodiversity loss can have important consequences for ecosystem functions, as exemplified by a large body of literature spanning at least three decades [1–3]. While connections between species diversity and ecosystem functions are now well-defined and understood, the importance of diversity within species is more elusive. Despite a surge in theoretical work on how intraspecific diversity can affect coexistence in simple community types [4,5], not much is known about how intraspecific diversity drives ecosystem processes in more complex community types. One particular challenge is that intraspecific diversity can be expressed as observable variation of functional traits, or instead subsist as genetic variation of which the consequences for ecosystem processes are harder to grasp.
Raffard et al. [6] examined how intraspecific biodiversity loss in a consumer fish changes species diversity at lower trophic levels and ecosystem processes in pond mesocosms. An interesting feature of this experiment is that it crosses functional and genetic intraspecific diversity. To do so, Raffard and colleagues measured and genotyped European minnow (P. phoxinus) individuals sampled from streams across southern France. Combining these collected specimens into experimental ponds allowed them to control functional (population variance of body size) and genetic intraspecific richness (number of genotypes).
Effects on minnow biomass production were mostly small; biomass was significantly reduced only when lowering both functional and genetic richness. However, the consequences for lower trophic levels (zooplankton and macroinvertebrates) were more pronounced and – importantly – not intuitive. For instance, the macroinvertebrate community was less species-diverse at higher minnow functional richness. If minnows with different body sizes would be the main regulator factors [7] explaining macroinvertebrate interactions, one would expect a more diverse set of minnow body sizes (i.e. higher functional minnow richness) to permit higher instead of lower macroinvertebrate richness. At the same time, the macroinvertebrate community was more species-diverse at higher minnow genotype richness, which could indicate unobserved minnow traits determining macroinvertebrate diversity more than the usual suspects (functional consumer richness). Such unobserved traits could be behavioral traits, allowing for resource partitioning among fish.
The consequences of functional minnow diversity loss on zooplankton diversity were negative, as expected in case body size differences among fish would facilitate coexistence of their zooplankton prey, as explained above. However, this was only the case when genetic diversity was high, suggesting nonstraightforward interactive effects of observed and non-observed traits on prey diversity.
The effects of functional and genetic minnow diversity loss on invertebrate (macroinvertebrates and zooplankton) abundance were more consistent than for invertebrate diversity. This suggests again nonstraightforward relationships in this experimental ecosystem, but now between invertebrate diversity and abundance. When using abundance as a proxy for an ecosystem process (which the authors did not), this result illustrates that biodiversity loss in multitrophic communities can have consequences that are challenging to interpret, let alone predict [8,9]. Path analyses showed how the observed changes of invertebrate diversity and abundance co-determined decomposition, a key ecosystem function. These path analyses had highest explanatory power show when including both kinds of intraspecific diversity.
Taken together, the results by Raffard and colleagues suggest that genetic consumer richness can drive species diversity of connected trophic levels and ecosystem processes with similar magnitude as functional diversity. Indeed, the effects of genetic consumer richness were shown to be so strong as to compensate or exacerbate the loss of observed functional richness. The exact mechanisms explaining these effects remain to be identified, however. The possibility that fish grazing by fish with different (observed or not observed) traits regulates coexistence among invertebrate prey, for instance, would depend on how strong fish consumption feeds back on prey growth during a 30-week experiment. As the authors indicate, detailed studies on resource partitioning among consumers (e.g. using stable isotope labelling) can shed light on these matters. Doing so may address a more fundamental question, which is if the mechanisms linking intraspecific diversity to function are different from those linking interspecific diversity to function, and at what time scales.
References
[1] Tilman D, Downing JA (1994) Biodiversity and stability in grasslands. Nature, 367, 363–365. https://doi.org/10.1038/367363a0
[2] Cardinale BJ, Duffy JE, Gonzalez A, Hooper DU, Perrings C, Venail P, Narwani A, Mace GM, Tilman D, Wardle DA, Kinzig AP, Daily GC, Loreau M, Grace JB, Larigauderie A, Srivastava DS, Naeem S (2012) Biodiversity loss and its impact on humanity. Nature, 486, 59–67. https://doi.org/10.1038/nature11148
[3] De Laender F, Rohr JR, Ashauer R, Baird DJ, Berger U, Eisenhauer N, Grimm V, Hommen U, Maltby L, Meliàn CJ, Pomati F, Roessink I, Radchuk V, Brink PJV den (2016) Reintroducing Environmental Change Drivers in Biodiversity–Ecosystem Functioning Research. Trends in Ecology & Evolution, 31, 905–915. https://doi.org/10.1016/j.tree.2016.09.007
[4] Hart SP, Schreiber SJ, Levine JM (2016) How variation between individuals affects species coexistence. Ecology Letters, 19, 825–838. https://doi.org/10.1111/ele.12618
[5] Barabás G, D’Andrea R (2016) The effect of intraspecific variation and heritability on community pattern and robustness. Ecology Letters, 19, 977–986. https://doi.org/10.1111/ele.12636
[6] Raffard A, Cucherousset J, Montoya JM, Richard M, Acoca-Pidolle S, Poésy C, Garreau A, Santoul F, Blanchet S (2020) Intraspecific diversity loss in a predator species alters prey community structure and ecosystem functions. bioRxiv, 2020.06.10.144337, ver. 3 peer-reviewed and recommended by PCI Ecology. https://doi.org/10.1101/2020.06.10.144337
[7] Pásztor L, Botta-Dukát Z, Magyar G, Czárán T, Meszéna G. Theory-Based Ecology: A Darwinian approach. Oxford University Press. https://doi.org/10.1093/acprof:oso/9780199577859.001.0001
[8] Binzer A, Guill C, Rall BC, Brose U (2016) Interactive effects of warming, eutrophication and size structure: impacts on biodiversity and food-web structure. Global Change Biology, 22, 220–227. https://doi.org/10.1111/gcb.13086
[9] Schwarz B, Barnes AD, Thakur MP, Brose U, Ciobanu M, Reich PB, Rich RL, Rosenbaum B, Stefanski A, Eisenhauer N (2017) Warming alters energetic structure and function but not resilience of soil food webs. Nature Climate Change, 7, 895–900. https://doi.org/10.1038/s41558-017-0002-z
| Intraspecific diversity loss in a predator species alters prey community structure and ecosystem functions | Allan Raffard, Julien Cucherousset, José M. Montoya, Murielle Richard, Samson Acoca-Pidolle, Camille Poésy, Alexandre Garreau, Frédéric Santoul & Simon Blanchet. | <p>Loss in intraspecific diversity can alter ecosystem functions, but the underlying mechanisms are still elusive, and intraspecific biodiversity-ecosystem function relationships (iBEF) have been restrained to primary producers. Here, we manipulat... | 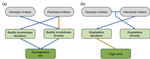 | Community ecology, Ecosystem functioning, Experimental ecology, Food webs, Freshwater ecology | Frederik De Laender | Andrew Barnes | 2020-06-15 09:04:53 | View |
Uncertain predictions of species responses to perturbations lead to underestimate changes at ecosystem level in diverse systems
Recommended by Elisa Thebault based on reviews by Carlos Melian and 1 anonymous reviewer
Different sources of uncertainty are known to affect our ability to predict ecological dynamics (Petchey et al. 2015). However, the consequences of uncertainty on prediction biases have been less investigated, especially when predictions are scaled up to higher levels of organisation as is commonly done in ecology for instance. The study of Orr et al. (2020) addresses this issue. It shows that, in complex systems, the uncertainty of unbiased predictions at a lower level of organisation (e.g. species level) leads to a bias towards underestimation of change at higher level of organisation (e.g. ecosystem level). This bias is strengthened by larger uncertainty and by higher dimensionality of the system.
This general result has large implications for many fields of science, from economics to energy supply or demography. In ecology, as discussed in this study, these results imply that the uncertainty of predictions of species’ change increases the probability of underestimation of changes of diversity and stability at community and ecosystem levels, especially when species richness is high. The uncertainty of predictions of species’ change also increases the probability of underestimation of change when multiple ecosystem functions are considered at once, or when the combined effect of multiple stressors is considered.
The consequences of species diversity on ecosystem functions and stability have received considerable attention during the last decades (e.g. Cardinale et al. 2012, Kéfi et al. 2019). However, since the bias towards underestimation of change increases with species diversity, future studies will need to investigate how the general statistical effect outlined by Orr et al. might affect our understanding of the well-known relationships between species diversity and ecosystem functioning and stability in response to perturbations.
References
Cardinale BJ, Duffy JE, Gonzalez A, Hooper DU, Perrings C, Venail P, Narwani A, Mace GM, Tilman D, Wardle DA, Kinzig AP, Daily GC, Loreau M, Grace JB, Larigauderie A, Srivastava DS, Naeem S (2012) Biodiversity loss and its impact on humanity. Nature, 486, 59–67. https://doi.org/10.1038/nature11148
Kéfi S, Domínguez‐García V, Donohue I, Fontaine C, Thébault E, Dakos V (2019) Advancing our understanding of ecological stability. Ecology Letters, 22, 1349–1356. https://doi.org/10.1111/ele.13340
Orr JA, Piggott JJ, Jackson A, Arnoldi J-F (2020) Why scaling up uncertain predictions to higher levels of organisation will underestimate change. bioRxiv, 2020.05.26.117200. https://doi.org/10.1101/2020.05.26.117200
Petchey OL, Pontarp M, Massie TM, Kéfi S, Ozgul A, Weilenmann M, Palamara GM, Altermatt F, Matthews B, Levine JM, Childs DZ, McGill BJ, Schaepman ME, Schmid B, Spaak P, Beckerman AP, Pennekamp F, Pearse IS (2015) The ecological forecast horizon, and examples of its uses and determinants. Ecology Letters, 18, 597–611. https://doi.org/10.1111/ele.12443
| Why scaling up uncertain predictions to higher levels of organisation will underestimate change | James Orr, Jeremy Piggott, Andrew Jackson, Jean-François Arnoldi | <p>Uncertainty is an irreducible part of predictive science, causing us to over- or underestimate the magnitude of change that a system of interest will face. In a reductionist approach, we may use predictions at the level of individual system com... | 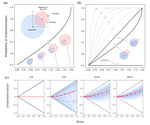 | Community ecology, Ecosystem functioning, Theoretical ecology | Elisa Thebault | Anonymous | 2020-06-02 15:41:12 | View |
Insect herbivory on urban trees: Complementary effects of tree neighbours and predation
Alex Stemmelen, Alain Paquette, Marie-Lise Benot, Yasmine Kadiri, Hervé Jactel, Bastien Castagneyrol
https://doi.org/10.1101/2020.04.15.042317
Tree diversity is associated with reduced herbivory in urban forest
Recommended by Ruth Arabelle Hufbauer and Ian Pearse based on reviews by Ian Pearse and Freerk Molleman
Urban ecology, the study of ecological systems in our increasingly urbanized world, is crucial to planning and redesigning cities to enhance ecosystem services (Kremer et al. 2016), human health and well-being and further conservation goals (Dallimer et al. 2012). Urban trees are a crucial component of urban streets and parks that provide shade and cooling through evapotranspiration (Fung and Jim 2019), improve air quality (Lai and Kontokosta 2019), help control storm water (Johnson and Handel 2016), and conserve wildlife (Herrmann et al. 2012; de Andrade et al. 2020).
Ideally, management of urban forests strikes a balance between maintaining the health of urban trees while retaining those organisms, such as herbivores, that connect a tree to the urban ecosystem. Herbivory by arthropods can substantially affect tree growth and reproduction (Whittaker and Warrington 1985), and so understanding factors that influence herbivory in urban forests is important to effective management. At the same time, herbivorous arthropods are important as key components of urban bird diets (Airola and Greco 2019) and provide a backyard glimpse at forest ecosystems in an increasingly built environment (Pearse 2019). Maintenance of arthropod predators may be one way to retain arthropods in urban forests while keeping detrimental outbreaks of herbivores in check. In “Insect herbivory on urban trees: Complementary effects of tree neighbors and predation” Stemmelen and colleagues (Stemmelen et al. 2020) use a clever sampling design to show that insect herbivory decreases as the diversity of neighboring trees increased. By placing artificial larvae out on trees, they provide evidence that increased predation in higher diversity urban forest patches might drive patterns in herbivory. The paper also demonstrates the importance of tree species identity in determining leaf herbivory.
The implications of this research for urban foresters is that deliberately planting diverse urban forests will help manage insect herbivores and should thus improve tree health. Potential knock-on effects could be seen for the ecosystem services provided by urban forests. While it might be tempting to simply plant more of the species that are subject to low current rates of herbivory, other research on the long-term vulnerability of monocultures to attack by specialist pathogens and herbivores (Tooker and Frank 2012) cautions against such an approach. Furthermore, the importance of urban forest insects to birds, including migrating birds, argues for managing urban forests more holistically (Greco and Airola 2018).
Stemmelen et al. (2020) used an observational approach focused on urban forests in Montreal, Canada in their research. Their findings suggest follow-up research focused on a broader cross-section of urban forests across latitudes, as well as experimental research. Experiments could, for example, exclude avian predators with netting (e.g. (Marquis and Whelan 1994)) to evaluate the relative importance of birds to managing urban insects on trees, as well as the flip side of that equation, the important to birds of insects on urban trees.
In summary, Stemmelen and colleague’s manuscript illustrates clever sampling and use of observational data to infer broader ecological patterns. It is worth reading to better understand the role of diversity in driving plant-insect community interactions and given the implications of the findings for sustainable long-term management of urban forests.
References
Airola, D. and Greco, S. (2019). Birds and oaks in California’s urban forest. Int. Oaks, 30, 109–116.
de Andrade, A.C., Medeiros, S. and Chiarello, A.G. (2020). City sloths and marmosets in Atlantic forest fragments with contrasting levels of anthropogenic disturbance. Mammal Res., 65, 481–491. doi: https://doi.org/10.1007/s13364-020-00492-0
Dallimer, M., Irvine, K.N., Skinner, A.M.J., Davies, Z.G., Rouquette, J.R., Maltby, L.L., et al. (2012). Biodiversity and the Feel-Good Factor: Understanding Associations between Self-Reported Human Well-being and Species Richness. Bioscience, 62, 47–55. doi: https://doi.org/10.1525/bio.2012.62.1.9
Fung, C.K.W. and Jim, C.Y. (2019). Microclimatic resilience of subtropical woodlands and urban-forest benefits. Urban For. Urban Green., 42, 100–112. doi: https://doi.org/10.1016/j.ufug.2019.05.014
Greco, S.E. and Airola, D.A. (2018). The importance of native valley oaks (Quercus lobata) as stopover habitat for migratory songbirds in urban Sacramento, California, USA. Urban For. Urban Green., 29, 303–311. doi: https://doi.org/10.1016/j.ufug.2018.01.005
Herrmann, D.L., Pearse, I.S. and Baty, J.H. (2012). Drivers of specialist herbivore diversity across 10 cities. Landsc. Urban Plan., 108, 123–130. doi: https://doi.org/10.1016/j.landurbplan.2012.08.007
Johnson, L.R. and Handel, S.N. (2016). Restoration treatments in urban park forests drive long-term changes in vegetation trajectories. Ecol. Appl., 26, 940–956. doi: https://doi.org/10.1890/14-2063
Kremer, P., Hamstead, Z., Haase, D., McPhearson, T., Frantzeskaki, N., Andersson, E., et al. (2016). Key insights for the future of urban ecosystem services research. Ecol. Soc., 21: 29. doi: http://doi.org/10.5751/ES-08445-210229
Lai, Y. and Kontokosta, C.E. (2019). The impact of urban street tree species on air quality and respiratory illness: A spatial analysis of large-scale, high-resolution urban data. Heal. Place, 56, 80–87. doi: https://doi.org/10.1016/j.healthplace.2019.01.016
Marquis, R.J. and Whelan, C.J. (1994). Insectivorous birds increase growth of white oak through consumption of leaf-chewing insects. Ecology, 75, 2007–2014. doi: https://doi.org/10.2307/1941605
Pearse, I.S. (2019). Insect herbivores on urban native oak trees. Int. Oaks, 30, 101–108.
Stemmelen, A., Paquette, A., Benot, M.-L., Kadiri, Y., Jactel, H. and Castagneyrol, B. (2020) Insect herbivory on urban trees: Complementary effects of tree neighbours and predation. bioRxiv, 2020.04.15.042317, ver. 5 peer-reviewed and recommended by PCI Ecology. doi: https://doi.org/10.1101/2020.04.15.042317
Tooker, J. F., and Frank, S. D. (2012). Genotypically diverse cultivar mixtures for insect pest management and increased crop yields. J. Appl. Ecol., 49(5), 974-985. doi: https://doi.org/10.1111/j.1365-2664.2012.02173.x
Whittaker, J.B. and Warrington, S. (1985). An experimental field study of different levels of insect herbivory induced By Formica rufa predation on Sycamore (Acer pseudoplatanus) III. Effects on Tree Growth. J. Appl. Ecol., 22, 797. doi: https://doi.org/10.2307/2403230
| Insect herbivory on urban trees: Complementary effects of tree neighbours and predation | Alex Stemmelen, Alain Paquette, Marie-Lise Benot, Yasmine Kadiri, Hervé Jactel, Bastien Castagneyrol | <p>Insect herbivory is an important component of forest ecosystems functioning and can affect tree growth and survival. Tree diversity is known to influence insect herbivory in natural forest, with most studies reporting a decrease in herbivory wi... | 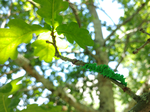 | Biodiversity, Biological control, Community ecology, Ecosystem functioning, Herbivory | Ruth Arabelle Hufbauer | | 2020-04-20 13:49:36 | View |