Latest recommendations
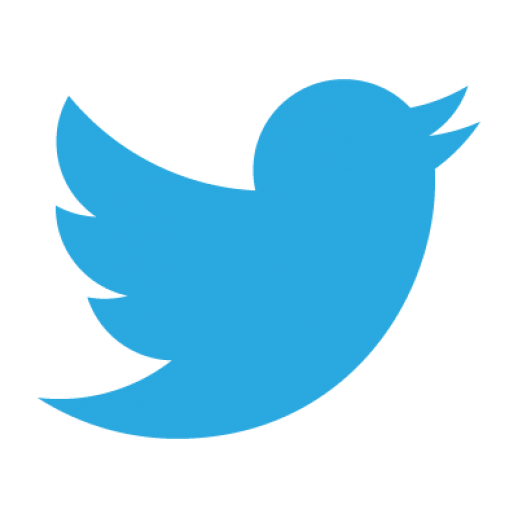
Id | Title | Authors | Abstract▲ | Picture | Thematic fields | Recommender | Reviewers | Submission date | |
---|---|---|---|---|---|---|---|---|---|
28 Apr 2023
Most diverse, most neglected: weevils (Coleoptera: Curculionoidea) are ubiquitous specialized brood-site pollinators of tropical floraJulien Haran, Gael J. Kergoat, Bruno A. S. de Medeiros https://hal.inrae.fr/hal-03780127Pollination-herbivory by weevils claiming for recognition: the Cinderella among pollinatorsRecommended by Juan Arroyo based on reviews by Susan Kirmse, Carlos Eduardo Nunes and 2 anonymous reviewersSince Charles Darwin times, and probably earlier, naturalists have been eager to report the rarest pollinators being discovered, and this still happens even in recent times; e.g., increased evidence of lizards, cockroaches, crickets or earwigs as pollinators (Suetsugu 2018, Komamura et al. 2021, de Oliveira-Nogueira et al. 2023), shifts to invasive animals as pollinators, including passerine birds and rats (Pattemore & Wilcove 2012), new amazing cases of mimicry in pollination, such as “bleeding” flowers that mimic wounded insects (Heiduk et al., 2023) or even the possibility that a tree frog is reported for the first time as a pollinator (de Oliveira-Nogueira et al. 2023). This is in part due to a natural curiosity of humans about rarity, which pervades into scientific insight (Gaston 1994). Among pollinators, the apparent rarity of some interaction types is sometimes a symptom of a lack of enough inquiry. This seems to be the case of weevil pollination, given that these insects are widely recognized as herbivores, particularly those that use plant parts to nurse their breed and never were thought they could act also as mutualists, pollinating the species they infest. This is known as a case of brood site pollination mutualism (BSPM), which also involves an antagonistic counterpart (herbivory) to which plants should face. This is the focus of the manuscript (Haran et al. 2023) we are recommending here. There is wide treatment of this kind of pollination in textbooks, albeit focused on yucca-yucca moth and fig-fig wasp interactions due to their extreme specialization (Pellmyr 2003, Kjellberg et al. 2005), and more recently accompanied by Caryophyllaceae-moth relationship (Kephart et al. 2006). Here we find a detailed review that shows that the most diverse BSPM, in terms of number of plant and pollinator species involved, is that of weevils in the tropics. The mechanism of BSPM does not involve a unique morphological syndrome, as it is mostly functional and thus highly dependent on insect biology (Fenster & al. 2004), whereas the flower phenotypes are highly divergent among species. Probably, the inconspicuous nature of the interaction, and the overwhelming role of weevils as seed predators, even as pests, are among the causes of the neglection of weevils as pollinators, as it could be in part the case of ants as pollinators (de Vega et al. 2014). The paper by Haran et al (2023) comes to break this point. Thus, the rarity of weevil pollination in former reports is not a consequence of an anecdotical nature of this interaction, even for the BSPM, according to the number of cases the authors are reporting, both in terms of plant and pollinator species involved. This review has a classical narrative format which involves a long text describing the natural history behind the cases. It is timely and fills the gap for this important pollination interaction for biodiversity and also for economic implications for fruit production of some crops. Former reviews have addressed related topics on BSPM but focused on other pollinators, such as those mentioned above. Besides, the review put much effort into the animal side of the interaction, which is not common in the pollination literature. Admittedly, the authors focus on the detailed description of some paradigmatic cases, and thereafter suggest that these can be more frequently reported in the future, based on varied evidence from morphology, natural history, ecology, and distribution of alleged partners. This procedure was common during the development of anthecology, an almost missing term for floral ecology (Baker 1983), relying on accumulative evidence based on detailed observations and experiments on flowers and pollinators. Currently, a quantitative approach based on the tools of macroecological/macroevolutionary analyses is more frequent in reviews. However, this approach requires a high amount of information on the natural history of the partnership, which allows for sound hypothesis testing. By accumulating this information, this approach allows the authors to pose specific questions and hypotheses which can be tested, particularly on the efficiency of the systems and their specialization degree for both the plants and the weevils, apparently higher for the latter. This will guarantee that this paper will be frequently cited by floral ecologists and evolutionary biologists and be included among the plethora of floral syndromes already described, currently based on more explicit functional grounds (Fenster et al. 2004). In part, this is one of the reasons why the sections focused on future prospects is so large in the review. I foresee that this mutualistic/antagonistic relationship will provide excellent study cases for the relative weight of these contrary interactions among the same partners and its relationship with pollination specialization-generalization and patterns of diversification in the plants and/or the weevils. As new studies are coming, it is possible that BSPM by weevils appears more common in non-tropical biogeographical regions. In fact, other BSPM are not so uncommon in other regions (Prieto-Benítez et al. 2017). In the future, it would be desirable an appropriate testing of the actual effect of phylogenetic niche conservatism, using well known and appropriately selected BSPM cases and robust phylogenies of both partners in the mutualism. Phylogenetic niche conservatism is a central assumption by the authors to report as many cases as possible in their review, and for that they used taxonomic relatedness. As sequence data and derived phylogenies for large numbers of vascular plant species are becoming more frequent (Jin & Quian 2022), I would recommend the authors to perform a comparative analysis using this phylogenetic information. At least, they have included information on phylogenetic relatedness of weevils involved in BSPM which allow some inferences on the multiple origins of this interaction. This is a good start to explore the drivers of these multiple origins through the lens of comparative biology. References Baker HG (1983) An Outline of the History of Anthecology, or Pollination Biology. In: L Real (ed). Pollination Biology. Academic Press. de-Oliveira-Nogueira CH, Souza UF, Machado TM, Figueiredo-de-Andrade CA, Mónico AT, Sazima I, Sazima M, Toledo LF (2023). Between fruits, flowers and nectar: The extraordinary diet of the frog Xenohyla truncate. Food Webs 35: e00281. https://doi.org/10.1016/j.fooweb.2023.e00281 Fenster CB W, Armbruster S, Wilson P, Dudash MR, Thomson JD (2004). Pollination syndromes and floral specialization. Annu. Rev. Ecol. Evol. Syst. 35: 375–403. https://doi.org/10.1146/annurev.ecolsys.34.011802.132347 Gaston KJ (1994). What is rarity? In KJ Gaston (ed): Rarity. Population and Community Biology Series, vol 13. Springer, Dordrecht. https://doi.org/10.1007/978-94-011-0701-3_1 Haran J, Kergoat GJ, Bruno, de Medeiros AS (2023) Most diverse, most neglected: weevils (Coleoptera: Curculionoidea) are ubiquitous specialized brood-site pollinators of tropical flora. hal. 03780127, version 2 peer-reviewed and recommended by Peer Community in Ecology. https://hal.inrae.fr/hal-03780127 Heiduk A, Brake I, Shuttleworth A, Johnson SD (2023) ‘Bleeding’ flowers of Ceropegia gerrardii (Apocynaceae-Asclepiadoideae) mimic wounded insects to attract kleptoparasitic fly pollinators. New Phytologist. https://doi.org/10.1111/nph.18888 Jin, Y., & Qian, H. (2022). V. PhyloMaker2: An updated and enlarged R package that can generate very large phylogenies for vascular plants. Plant Diversity, 44(4), 335-339. https://doi.org/10.1016/j.pld.2022.05.005 Kjellberg F, Jousselin E, Hossaert-Mckey M, Rasplus JY (2005). Biology, ecology, and evolution of fig-pollinating wasps (Chalcidoidea, Agaonidae). In: A. Raman et al (eds) Biology, ecology and evolution of gall-inducing arthropods 2, 539-572. Science Publishers, Enfield. Komamura R, Koyama K, Yamauchi T, Konno Y, Gu L (2021). Pollination contribution differs among insects visiting Cardiocrinum cordatum flowers. Forests 12: 452. https://doi.org/10.3390/f12040452 Pattemore DE, Wilcove DS (2012) Invasive rats and recent colonist birds partially compensate for the loss of endemic New Zealand pollinators. Proc. R. Soc. B 279: 1597–1605. https://doi.org/10.1098/rspb.2011.2036 Pellmyr O (2003) Yuccas, yucca moths, and coevolution: a review. Ann. Missouri Bot. Gard. 90: 35-55. https://doi.org/10.2307/3298524 Prieto-Benítez S, Yela JL, Giménez-Benavides L (2017) Ten years of progress in the study of Hadena-Caryophyllaceae nursery pollination. A review in light of new Mediterranean data. Flora, 232, 63-72. https://doi.org/10.1016/j.flora.2017.02.004 Suetsugu K (2019) Social wasps, crickets and cockroaches contribute to pollination of the holoparasitic plant Mitrastemon yamamotoi (Mitrastemonaceae) in southern Japan. Plant Biology 21 176–182. https://doi.org/10.1111/plb.12889 | Most diverse, most neglected: weevils (Coleoptera: Curculionoidea) are ubiquitous specialized brood-site pollinators of tropical flora | Julien Haran, Gael J. Kergoat, Bruno A. S. de Medeiros | <p style="text-align: justify;">In tropical environments, and especially tropical rainforests, a major part of pollination services is provided by diverse insect lineages. Unbeknownst to most, beetles, and more specifically hyperdiverse weevils (C... | Biodiversity, Evolutionary ecology, Pollination, Tropical ecology | Juan Arroyo | 2022-09-28 11:54:37 | View | ||
12 Apr 2023
![]() Feeding and growth variations affect δ13C and δ15N budgets during ontogeny in a lepidopteran larvaSamuel M. Charberet, Annick Maria, David Siaussat, Isabelle Gounand, Jérôme Mathieu https://doi.org/10.1101/2022.11.09.515573Refining our understanding how nutritional conditions affect 13C and 15N isotopic fractionation during ontogeny in a herbivorous insectRecommended by Gregor Kalinkat based on reviews by Anton Potapov and 1 anonymous reviewerUsing stable isotope fractionation to disentangle and understand the trophic positions of animals within the food webs they are embedded within has a long tradition in ecology (Post, 2002; Scheu, 2002). Recent years have seen increasing application of the method with several recent reviews summarizing past advancements in this field (e.g. Potapov et al., 2019; Quinby et al., 2020). In their new manuscript, Charberet and colleagues (2023) set out to refine our understanding of the processes that lead to nitrogen and carbon stable isotope fractionation by investigating how herbivorous insect larvae (specifically, the noctuid moth Spodoptera littoralis) respond to varying nutritional conditions (from starving to ad libitum feeding) in terms of stable isotopes enrichment. Though the underlying mechanisms have been experimentally investigated before in terrestrial invertebrates (e.g. in wolf spiders; Oelbermann & Scheu, 2002), the elegantly designed and adequately replicated experiments by Charberet and colleagues add new insights into this topic. Particularly, the authors provide support for the hypotheses that (A) 15N is disproportionately accumulated under fast growth rates (i.e. when fed ad libitum) and that (B) 13C is accumulated under low growth rates and starvation due to depletion of 13C-poor fat tissues. Applying this knowledge to field samples where feeding conditions are usually not known in detail is not straightforward, but the new findings could still help better interpretation of field data under specific conditions that make starvation for herbivores much more likely (e.g. droughts). Overall this study provides important methodological advancements for a better understanding of plant-herbivore interactions in a changing world. REFERENCES Charberet, S., Maria, A., Siaussat, D., Gounand, I., & Mathieu, J. (2023). Feeding and growth variations affect δ13C and δ15N budgets during ontogeny in a lepidopteran larva. bioRxiv, ver. 3 peer-reviewed and recommended by Peer Community in Ecology. https://doi.org/10.1101/2022.11.09.515573 Oelbermann, K., & Scheu, S. (2002). Stable Isotope Enrichment (δ 15N and δ 13C) in a Generalist Predator (Pardosa lugubris, Araneae: Lycosidae): Effects of Prey Quality. Oecologia, 130(3), 337–344. https://doi.org/10.1007/s004420100813 Post, D. M. (2002). Using stable isotopes to estimate trophic position: Models, methods, and assumptions. Ecology, 83(3), 703–718. https://doi.org/10.1890/0012-9658(2002)083[0703:USITET]2.0.CO;2 Potapov, A. M., Tiunov, A. V., & Scheu, S. (2019). Uncovering trophic positions and food resources of soil animals using bulk natural stable isotope composition. Biological Reviews, 94(1), 37–59. https://doi.org/10.1111/brv.12434 Quinby, B. M., Creighton, J. C., & Flaherty, E. A. (2020). Stable isotope ecology in insects: A review. Ecological Entomology, 45(6), 1231–1246. https://doi.org/10.1111/een.12934 Scheu, S. (2002). The soil food web: Structure and perspectives. European Journal of Soil Biology, 38(1), 11–20. https://doi.org/10.1016/S1164-5563(01)01117-7 | Feeding and growth variations affect δ13C and δ15N budgets during ontogeny in a lepidopteran larva | Samuel M. Charberet, Annick Maria, David Siaussat, Isabelle Gounand, Jérôme Mathieu | <p style="text-align: justify;">Isotopes are widely used in ecology to study food webs and physiology. The fractionation observed between trophic levels in nitrogen and carbon isotopes, explained by isotopic biochemical selectivity, is subject to ... | ![]() | Experimental ecology, Food webs, Physiology | Gregor Kalinkat | 2022-11-16 15:23:31 | View | |
11 Mar 2024
Sex differences in the relationship between maternal and neonate cortisol in a free-ranging large mammalAmin, B., Fishman, R., Quinn, M., Matas, D., Palme, R., Koren, L., Ciuti, S. https://doi.org/10.1101/2023.05.04.538920Stress and stress hormones’ transmission from mothers to offspringRecommended by Matthieu PaquetIndividuals can respond to environmental changes that they undergo directly (within-generation plasticity) but also through transgenerational plasticity, providing lasting effects that are transmitted to the next generations (Donelson et al. 2012; Munday et al. 2013; Kuijper & Hoyle 2015; Auge et al. 2017, Tariel et al. 2020). These parental effects can affect offspring via various mechanisms, notably via maternal transmission of hormones to the eggs or growing embryos (Mousseau & Fox 1998). While the effects of environmental quality may simply carry-over to the next generation (e.g., females in stressful environments give birth to offspring in poorer condition), parental effects may also be a mechanism that adjusts offspring phenotype in response to environmental variation and predictability, and thereby match offspring's phenotype to future environmental conditions (Gluckman et al. 2005; Marshall & Uller 2007; Dey et al. 2016; Yin et al. 2019), for example by preparing their offspring to an expected stressful environment. When females experience stress during gestation or egg formation, elevations in glucocorticoids (GC) are expected to affect offspring phenotype in many ways, from the offspring's own GC levels, to their growth and survival (Sheriff et al. 2017). This is a well established idea, but how strong is the evidence for this? A meta-analysis on birds found no clear effect of corticosterone manipulation on offspring traits (38 studies on 9 bird species for corticosterone manipulation; Podmokła et al. 2018). Another meta-analysis including 14 vertebrate species found no clear effect of prenatal stress on offspring GC (Thayer et al. 2018). Finally, a meta-analysis on wild vertebrates (23 species) found no clear effect of GC-mediated maternal effects on offspring traits (MacLeod et al. 2021). As often when facing such inconclusive results, context dependence has been suggested as one potential reason for such inconsistencies, for exemple sex specific effects (Groothuis et al. 2019, 2020). However, sex specific measures on offspring are scarce (Podmokła et al. 2018). Moreover, the literature available is still limited to a few, mostly “model” species. With their study, Amin et al. (2024) show the way to improve our understanding on GC transmission from mother to offspring and its effects in several aspects. First they used innovative non-invasive methods (which could broaden the range of species available to study) by quantifying cortisol metabolites from faecal samples collected from pregnant females, as proxy for maternal GC level, and relating it to GC levels from hairs of their neonate offspring. Second they used a free ranging large mammal (taxa from which literature is missing): the fallow deer (Dama dama). Third, they provide sex specific measures of GC levels. And finally but importantly, they are exemplary in their transparency regarding 1) the exploratory nature of their study, 2) their statistical thinking and procedure, and 3) the study limitations (e.g., low sample size and high within individual variation of measurements). I hope this study will motivate more research (on the fallow deer, and on other species) to broaden and strengthen our understanding of sex specific effects of maternal stress and CG levels on offspring phenotype and fitness. References Amin, B., Fishman, R., Quinn, M., Matas, D., Palme, R., Koren, L., & Ciuti, S. (2024). Sex differences in the relationship between maternal and foetal glucocorticoids in a free-ranging large mammal. bioRxiv, ver. 4 peer-reviewed and recommended by Peer Community in Ecology. https://doi.org/10.1101/2023.05.04.538920 Auge, G.A., Leverett, L.D., Edwards, B.R. & Donohue, K. (2017). Adjusting phenotypes via within-and across-generational plasticity. New Phytologist, 216, 343–349. https://doi.org/10.1111/nph.14495 Dey, S., Proulx, S.R. & Teotonio, H. (2016). Adaptation to temporally fluctuating environments by the evolution of maternal effects. PLoS biology, 14, e1002388. https://doi.org/10.1371/journal.pbio.1002388 Donelson, J.M., Munday, P.L., McCormick, M.I. & Pitcher, C.R. (2012). Rapid transgenerational acclimation of a tropical reef fish to climate change. Nature Climate Change, 2, 30. https://doi.org/10.1038/nclimate1323 Gluckman, P.D., Hanson, M.A. & Spencer, H.G. (2005). Predictive adaptive responses and human evolution. Trends in ecology & evolution, 20, 527–533. https://doi.org/10.1016/j.tree.2005.08.001 Groothuis, Ton GG, Bin-Yan Hsu, Neeraj Kumar, and Barbara Tschirren. "Revisiting mechanisms and functions of prenatal hormone-mediated maternal effects using avian species as a model." Philosophical Transactions of the Royal Society B 374, no. 1770 (2019): 20180115. https://doi.org/10.1098/rstb.2018.0115 Groothuis, Ton GG, Neeraj Kumar, and Bin-Yan Hsu. "Explaining discrepancies in the study of maternal effects: the role of context and embryo." Current Opinion in Behavioral Sciences 36 (2020): 185-192. https://doi.org/10.1016/j.cobeha.2020.10.006 Kuijper, B. & Hoyle, R.B. (2015). When to rely on maternal effects and when on phenotypic plasticity? Evolution, 69, 950–968. https://doi.org/10.1111/evo.12635 MacLeod, Kirsty J., Geoffrey M. While, and Tobias Uller. "Viviparous mothers impose stronger glucocorticoid‐mediated maternal stress effects on their offspring than oviparous mothers." Ecology and Evolution 11, no. 23 (2021): 17238-17259. Marshall, D.J. & Uller, T. (2007). When is a maternal effect adaptive? Oikos, 116, 1957–1963. https://doi.org/10.1111/j.2007.0030-1299.16203.x Mousseau, T.A. & Fox, C.W. (1998). Maternal effects as adaptations. Oxford University Press. Munday, P.L., Warner, R.R., Monro, K., Pandolfi, J.M. & Marshall, D.J. (2013). Predicting evolutionary responses to climate change in the sea. Ecology Letters, 16, 1488–1500. https://doi.org/10.1111/ele.12185 Podmokła, Edyta, Szymon M. Drobniak, and Joanna Rutkowska. "Chicken or egg? Outcomes of experimental manipulations of maternally transmitted hormones depend on administration method–a meta‐analysis." Biological Reviews 93, no. 3 (2018): 1499-1517. https://doi.org/10.1111/brv.12406 Sheriff, M. J., Bell, A., Boonstra, R., Dantzer, B., Lavergne, S. G., McGhee, K. E., MacLeod, K. J., Winandy, L., Zimmer, C., & Love, O. P. (2017). Integrating ecological and evolutionary context in the study of maternal stress. Integrative and Comparative Biology, 57(3), 437–449. https://doi.org/10.1093/icb/icx105 Tariel, Juliette, Sandrine Plénet, and Émilien Luquet. "Transgenerational plasticity in the context of predator-prey interactions." Frontiers in Ecology and Evolution 8 (2020): 548660. https://doi.org/10.3389/fevo.2020.548660 Thayer, Zaneta M., Meredith A. Wilson, Andrew W. Kim, and Adrian V. Jaeggi. "Impact of prenatal stress on offspring glucocorticoid levels: A phylogenetic meta-analysis across 14 vertebrate species." Scientific Reports 8, no. 1 (2018): 4942. https://doi.org/10.1038/s41598-018-23169-w Yin, J., Zhou, M., Lin, Z., Li, Q.Q. & Zhang, Y.-Y. (2019). Transgenerational effects benefit offspring across diverse environments: a meta-analysis in plants and animals. Ecology letters, 22, 1976–1986. https://doi.org/10.1111/ele.13373 | Sex differences in the relationship between maternal and neonate cortisol in a free-ranging large mammal | Amin, B., Fishman, R., Quinn, M., Matas, D., Palme, R., Koren, L., Ciuti, S. | <p style="text-align: justify;">Maternal phenotypes can have long-term effects on offspring phenotypes. These maternal effects may begin during gestation, when maternal glucocorticoid (GC) levels may affect foetal GC levels, thereby having an orga... | Evolutionary ecology, Maternal effects, Ontogeny, Physiology, Zoology | Matthieu Paquet | 2023-06-05 09:06:56 | View | ||
12 Jan 2024
![]() Methods for tagging an ectoparasite, the salmon louse Lepeophtheirus salmonisAlexius Folk, Adele Mennerat https://doi.org/10.1101/2023.08.31.555695Marking invertebrates using RFID tagsRecommended by Nicolas SchtickzelleGuiding and monitoring the efficiency of conservation efforts needs robust scientific background information, of which one key element is estimating wildlife abundance and its spatial and temporal variation. As raw counts are by nature incomplete counts of a population, correcting for detectability is required (Clobert, 1995; Turlure et al., 2018). This can be done with Capture-Mark-Recapture protocols (Iijima, 2020). Techniques for marking individuals are diverse, e.g. writing on butterfly wings, banding birds, or using natural specific patterns in the individual’s body such as leopard fur or whale tail. Advancement in technology opens new opportunities for developing marking techniques, including strategies to limit mark identification errors (Burchill & Pavlic, 2019), and for using active marks that can transmit data remotely or be read automatically. The details of such methodological developments frequently remain unpublished, the method being briefly described in studies that use it. For a few years, there has been however a renewed interest in proper publishing of methods for ecology and evolution. This study by Folk & Mennerat (2023) fits in this context, offering a nice example of detailed description and testing of a method to mark salmon ectoparasites using RFID tags. Such tags are extremely small, yet easy to use, even with automatic recording procedure. The study provides a very good basis protocol that should help researchers working for small species, in particular invertebrates. The study is complemented by a video illustrating the placement of the tag so the reader who would like to replicate the procedure can get a very precise idea of it. References Burchill, A. T., & Pavlic, T. P. (2019). Dude, where’s my mark? Creating robust animal identification schemes informed by communication theory. Animal Behaviour, 154, 203–208. https://doi.org/10.1016/j.anbehav.2019.05.013 Clobert, J. (1995). Capture-recapture and evolutionary ecology: A difficult wedding ? Journal of Applied Statistics, 22(5–6), 989–1008. Folk, A., & Mennerat, A. (2023). Methods for tagging an ectoparasite, the salmon louse Lepeophtheirus salmonis (p. 2023.08.31.555695). bioRxiv, ver. 2 peer-reviewed and recommended by Peer Community in Ecology. https://doi.org/10.1101/2023.08.31.555695 Iijima, H. (2020). A Review of Wildlife Abundance Estimation Models: Comparison of Models for Correct Application. Mammal Study, 45(3), 177–188. https://doi.org/10.3106/ms2019-0082 Turlure, C., Pe’er, G., Baguette, M., & Schtickzelle, N. (2018). A simplified mark–release–recapture protocol to improve the cost effectiveness of repeated population size quantification. Methods in Ecology and Evolution, 9(3), 645–656. https://doi.org/10.1111/2041-210X.12900 | Methods for tagging an ectoparasite, the salmon louse *Lepeophtheirus salmonis* | Alexius Folk, Adele Mennerat | <p style="text-align: justify;">Monitoring individuals within populations is a cornerstone in evolutionary ecology, yet individual tracking of invertebrates and particularly parasitic organisms remains rare. To address this gap, we describe here a... | ![]() | Dispersal & Migration, Evolutionary ecology, Host-parasite interactions, Marine ecology, Parasitology, Terrestrial ecology, Zoology | Nicolas Schtickzelle | 2023-09-04 15:25:08 | View | |
20 Feb 2023
![]() Best organic farming deployment scenarios for pest control: a modeling approachThomas Delattre, Mohamed-Mahmoud Memah, Pierre Franck, Pierre Valsesia, Claire Lavigne https://doi.org/10.1101/2022.05.31.494006Towards model-guided organic farming expansion for crop pest managementRecommended by Sandrine CharlesReduce the impact the intensification of human activities has on the environmental is the challenge the humanity faces today, a major challenge that could be compared to climbing Everest without an oxygen supply. Indeed, over-population, pollution, burning fossil fuels, and deforestation are all evils which have had hugely detrimental effects on the environment such as climate change, soil erosion, poor air quality, and scarcity of drinking water to name but a few. In response to the ever-growing consumer demand, agriculture has intensified massively along with a drastic increase in the use of chemicals to ensure an adequate food supply while controlling crop pests. In this context, to address the disastrous effects of the intensive usage of pesticides on both human health and biodiversity, organic farming (OF) revealed as a miracle remedy with multiple benefits. Delattre et al. (2023) present a powerful modelling approach to decipher the crossed effects of the landscape structure and the OF expansion scenario on the pest abundance, both in organic and conventional (CF) crop fields. To this end, the authors ingeniously combined a grid-based landscape model with a spatially explicit predator-pest model. Based on an extensive in silico simulation process, they explore a diversity of landscape structures differing in their amount of semi-natural habitats (SHN) and in their fragmentation, to finally propose a ranking of various expansion scenarios according to the pest control methods in organic farming as well as to the pest and predators’ dissemination capacities. In total, 9 landscape structures (3 proportions of SHN x 3 fragmentation levels) were crossed with 3 expansion scenarios (RD = a random distribution of OF and CF in the grid; IP = isolated CF are converted; GP = CF within aggregates are converted), 4 pest management practices, 3 initial densities and 36 biological parameter combinations driving the predator’ and pest’s population dynamics. This exhaustive exploration of possible combinations of landscape and farming practices highlighted the main drivers of the various OF expansion scenarios, such as increased spillover of predators in isolated OF/CF fields, increased pest management efficiency in large patches of CF and the importance of the distance between OF and CF. In the end, this study brings to light the crucial role that landscape planning plays when OF practices have limited efficiency on pests. It also provides convincing arguments to the fact that converting to organic isolated CF as a priority seems to be the most promising scenario to limit pest densities in CF crops while improving predator to pest ratios (considered as a proxy of conservation biological control) in OF ones without increasing pest densities. Once further completed with model calibration validation based on observed life history traits data for both predators and pests, this work should be very helpful in sustaining policy makers to convince farmers of engaging in organic farming. REFERENCES Delattre T, Memah M-M, Franck P, Valsesia P, Lavigne C (2023) Best organic farming deployment scenarios for pest control: a modeling approach. bioRxiv, 2022.05.31.494006, ver. 2 peer-reviewed and recommended by Peer Community in Ecology. https://doi.org/10.1101/2022.05.31.494006 | Best organic farming deployment scenarios for pest control: a modeling approach | Thomas Delattre, Mohamed-Mahmoud Memah, Pierre Franck, Pierre Valsesia, Claire Lavigne | <p style="text-align: justify;">Organic Farming (OF) has been expanding recently around the world in response to growing consumer demand and as a response to environmental concerns. Its share of agricultural landscapes is expected to increase in t... | ![]() | Agroecology, Biological control, Landscape ecology | Sandrine Charles | 2022-06-03 11:41:14 | View | |
20 Feb 2024
![]() Functional trade-offs: exploring the temporal response of field margin plant communities to climate change and agricultural practicesIsis Poinas, Christine N Meynard, Guillaume Fried https://doi.org/10.1101/2023.03.03.530956Unravelling plant diversity in agricultural field margins in France: plant species better adapted to climate change need other agricultures to persistRecommended by Julia AstegianoAgricultural field margin plants, often referred to as “spontaneous” species, are key for the stabilization of several social-ecological processes related to crop production such as pollination or pest control (Tamburini et al. 2020). Because of its beneficial function, increasing the diversity of field margin flora becomes as important as crop diversity in process-based agricultures such as agroecology. Contrary, supply-dependent intensive agricultures produce monocultures and homogenized environments that might benefit their productivity, which generally includes the control or elimination of the field margin flora (Emmerson et al. 2016, Aligner 2018). Considering that different agricultural practices are produced by (and produce) different territories (Moore 2020) and that they are also been shaped by current climate change, we urgently need to understand how agricultural intensification constrains the potential of territories to develop agriculture more resilient to such change (Altieri et al., 2015). Thus, studies unraveling how agricultural practices' effects on agricultural field margin flora interact with those of climate change is of main importance, as plant strategies better adapted to such social-ecological processes may differ. References Alignier, A., 2018. Two decades of change in a field margin vegetation metacommunity as a result of field margin structure and management practice changes. Agric., Ecosyst. & Environ., 251, 1–10. https://doi.org/10.1016/j.agee.2017.09.013 Altieri, M.A., Nicholls, C.I., Henao, A., Lana, M.A., 2015. Agroecology and the design of climate change-resilient farming systems. Agron. Sustain. Dev. 35, 869–890. https://doi.org/10.1007/s13593-015-0285-2 Emmerson, M., Morales, M. B., Oñate, J. J., Batary, P., Berendse, F., Liira, J., Aavik, T., Guerrero, I., Bommarco, R., Eggers, S., Pärt, T., Tscharntke, T., Weisser, W., Clement, L. & Bengtsson, J. (2016). How agricultural intensification affects biodiversity and ecosystem services. In Adv. Ecol. Res. 55, 43-97. https://doi.org/10.1016/bs.aecr.2016.08.005 Grime, J. P., 1977. Evidence for the existence of three primary strategies in plants and its relevance to ecological and evolutionary theory. The American Naturalist, 111(982), 1169–1194. https://doi.org/10.1086/283244 Grime, J. P., 1988. The C-S-R model of primary plant strategies—Origins, implications and tests. In L. D. Gottlieb & S. K. Jain, Plant Evolutionary Biology (pp. 371–393). Springer Netherlands. https://doi.org/10.1007/978-94-009-1207-6_14 Moore, J., 2020. El capitalismo en la trama de la vida (Capitalism in The Web of Life). Traficantes de sueños, Madrid, Spain. Poinas, I., Fried, G., Henckel, L., & Meynard, C. N., 2023. Agricultural drivers of field margin plant communities are scale-dependent. Bas. App. Ecol. 72, 55-63. https://doi.org/10.1016/j.baae.2023.08.003 Poinas, I., Meynard, C. N., Fried, G., 2024. Functional trade-offs: exploring the temporal response of field margin plant communities to climate change and agricultural practices, bioRxiv, ver. 4 peer-reviewed and recommended by Peer Community in Ecology. https://doi.org/10.1101/2023.03.03.530956 Tamburini, G., Bommarco, R., Wanger, T.C., Kremen, C., Van Der Heijden, M.G., Liebman, M., Hallin, S., 2020. Agricultural diversification promotes multiple ecosystem services without compromising yield. Sci. Adv. 6, eaba1715. https://doi.org/10.1126/sciadv.aba1715 | Functional trade-offs: exploring the temporal response of field margin plant communities to climate change and agricultural practices | Isis Poinas, Christine N Meynard, Guillaume Fried | <p style="text-align: justify;">Over the past decades, agricultural intensification and climate change have led to vegetation shifts. However, functional trade-offs linking traits responding to climate and farming practices are rarely analyzed, es... | ![]() | Agroecology, Biodiversity, Botany, Climate change, Community ecology | Julia Astegiano | 2023-03-04 15:40:35 | View | |
29 Aug 2023
![]() Provision of essential resources as a persistence strategy in food websMichael Raatz https://doi.org/10.1101/2023.01.27.525839High-order interactions in food webs may strongly impact persistence of speciesRecommended by Cédric GaucherelMichael Raatz (2023) provides here a relevant exploration of higher-order interactions, i.e. interactions involving more than two related species (Terry et al. 2019), in the case of food web and competition interactions. More precisely, he shows by modeling that essential resources may significantly mediate focal species' persistence. Simultaneously, the provision of essential resources may strongly affect the resulting community structure, by driving to extinction first the predator and then, depending on the higher-order interaction, potentially also the associated competitor. Today, all ecologists should be aware of the potential effects of high-order interactions on species' (and likely on ecosystem's) fate (Golubski et al. 2016, Grilli et al. 2017). Yet, we should soon be prepared to include any high-order interaction into any interaction network (i.e. not only between species, but also between species and abiotic components, and between biotic, anthropogenic and abiotic components too). For this purpose, we will need innovative approaches such as hypergraphs (Golubski et al. 2016) and discrete-event models (Gaucherel and Pommereau 2019, Thomas et al. 2022) able to manage highly complex interactions, with numerous interacting components and variables. Such a rigorous study is a necessary and preliminary step in taking into account such a higher complexity. References Gaucherel, C. and F. Pommereau. 2019. Using discrete systems to exhaustively characterize the dynamics of an integrated ecosystem. Methods in Ecology and Evolution 00:1–13. https://doi.org/10.1111/2041-210X.13242 Golubski, A. J., E. E. Westlund, J. Vandermeer, and M. Pascual. 2016. Ecological Networks over the Edge: Hypergraph Trait-Mediated Indirect Interaction (TMII) Structure trends in Ecology & Evolution 31:344-354. https://doi.org/10.1016/j.tree.2016.02.006 Grilli, J., G. Barabas, M. J. Michalska-Smith, and S. Allesina. 2017. Higher-order interactions stabilize dynamics in competitive network models. Nature 548:210-213. https://doi.org/10.1038/nature23273 Raatz, M. 2023. Provision of essential resources as a persistence strategy in food webs. bioRxiv, ver. 3 peer-reviewed and recommended by Peer Community in Ecology. https://doi.org/10.1101/2023.01.27.525839 Terry, J. C. D., R. J. Morris, and M. B. Bonsall. 2019. Interaction modifications lead to greater robustness than pairwise non-trophic effects in food webs. Journal of Animal Ecology 88:1732-1742. https://doi.org/10.1111/1365-2656.13057 Thomas, C., M. Cosme, C. Gaucherel, and F. Pommereau. 2022. Model-checking ecological state-transition graphs. PLoS Computational Biology 18:e1009657. https://doi.org/10.1371/journal.pcbi.1009657 | Provision of essential resources as a persistence strategy in food webs | Michael Raatz | <p style="text-align: justify;">Pairwise interactions in food webs, including those between predator and prey are often modulated by a third species. Such higher-order interactions are important structural components of natural food webs that can ... | ![]() | Biodiversity, Coexistence, Competition, Ecological stoichiometry, Food webs, Interaction networks, Theoretical ecology | Cédric Gaucherel | 2023-02-23 17:48:26 | View | |
13 May 2023
![]() Symbiotic nutrient cycling enables the long-term survival of Aiptasia in the absence of heterotrophic food sourcesNils Radecker, Anders Meibom https://doi.org/10.1101/2022.12.07.519152Constraining the importance of heterotrophic vs autotrophic feeding in photosymbiotic cnidariansRecommended by Ulisse CardiniThe symbiosis with autotrophic dinoflagellate algae has enabled heterotrophic Cnidaria to thrive in nutrient-poor tropical waters (Muscatine and Porter 1977; Stanley 2006). In particular, mixotrophy, i.e. the ability to acquire nutrients through both autotrophy and heterotrophy, confers a competitive edge in oligotrophic waters, allowing photosymbiotic Cnidaria to outcompete benthic organisms limited to a single diet (e.g., McCook 2001). However, the relative importance of autotrophy vs heterotrophy in sustaining symbiotic cnidarian’s nutrition is still the subject of intense research. In fact, figuring out the cellular mechanisms by which symbiotic Cnidaria acquire a balanced diet for their metabolism and growth is relevant to our understanding of their physiology under varying environmental conditions and in response to anthropogenic perturbations. In this study's long-term starvation experiment, Radecker & Meibom (2023) investigated the survival of the photosymbiotic sea anemone Aiptasia in the absence of heterotrophic feeding. After one year of heterotrophic starvation, Apitasia anemones remained fully viable but showed an 85 % reduction in biomass. Using 13C-bicarbonate and 15N-ammonium labeling, electron microscopy and NanoSIMS imaging, the authors could clearly show that the contribution of algal-derived nutrients to the host metabolism remained unaffected as a result of increased algal photosynthesis and more efficient carbon translocation. At the same time, the absence of heterotrophic feeding caused severe nitrogen limitation in the starved Apitasia anemones. Overall, this study provides valuable insights into nutrient exchange within the symbiosis between Cnidaria and dinoflagellate algae at the cellular level and sheds new light on the importance of heterotrophic feeding as a nitrogen acquisition strategy for holobiont growth in oligotrophic waters. REFERENCES McCook L (2001) Competition between corals and algal turfs along a gradient of terrestrial influence in the nearshore central Great Barrier Reef. Coral Reefs 19:419–425. https://doi.org/10.1007/s003380000119 Muscatine L, Porter JW (1977) Reef corals: mutualistic symbioses adapted to nutrient-poor environments. Bioscience 27:454–460. https://doi.org/10.2307/1297526 Radecker N, Meibom A (2023) Symbiotic nutrient cycling enables the long-term survival of Aiptasia in the absence of heterotrophic food sources. bioRxiv, ver. 3 peer-reviewed and recommended by Peer Community in Ecology. https://doi.org/10.1101/2022.12.07.519152 Stanley GD Jr (2006) Photosymbiosis and the evolution of modern coral reefs. Science 312:857–858. https://doi.org/10.1126/science.1123701 | Symbiotic nutrient cycling enables the long-term survival of Aiptasia in the absence of heterotrophic food sources | Nils Radecker, Anders Meibom | <p style="text-align: justify;">Phototrophic Cnidaria are mixotrophic organisms that can complement their heterotrophic diet with nutrients assimilated by their algal endosymbionts. Metabolic models suggest that the translocation of photosynthates... | ![]() | Eco-evolutionary dynamics, Microbial ecology & microbiology, Symbiosis | Ulisse Cardini | 2022-12-12 10:50:55 | View | |
06 Nov 2023
![]() Influence of mimicry on extinction risk in Aculeata: a theoretical approachMaxime Boutin, Manon Costa, Colin Fontaine, Adrien Perrard, Violaine Llaurens https://doi.org/10.1101/2022.10.21.513153Mullerian and Batesian mimicry can influence population and community dynamicsRecommended by Amanda Franklin based on reviews by Jesus Bellver and 1 anonymous reviewerMimicry between species has long attracted the attention of scientists. Over a century ago, Bates first proposed that palatable species should gain a benefit by resembling unpalatable species (Bates 1862). Not long after, Müller suggested that there could also be a mutual advantage for two unpalatable species to mimic one another to reduce predator error (Müller 1879). These forms of mimicry, Batesian and Müllerian, are now widely studied, providing broad insights into behaviour, ecology and evolution. Numerous taxa, including both invertebrates and vertebrates, show examples of Batesian or Müllerian mimicry. Bees and wasps provide a particularly interesting case due to the differences in defence between females and males of the same species. While both males and females may display warning colours, only females can sting and inject venom to cause pain and allow escape from predators. Therefore, males are palatable mimics and can resemble females of their own species or females of another species (dual sex-limited mimicry). This asymmetry in defence could have impacts on both population structure and community assembly, yet research into mimicry largely focuses on systems without sex differences. Here, Boutin and colleagues (2023) use a differential equations model to explore the effect of mimicry on population structure and community assembly for sex-limited defended species. Specifically, they address three questions, 1) how do female noxiousness and sex-ratio influence the extinction risk of a single species?; 2) what is the effect of mimicry on species co-existence? and 3) how does dual sex-limited mimicry influence species co-existence? Their results reveal contexts in which populations with undefended males can persist, the benefit of Müllerian mimicry for species coexistence and that dual sex-limited mimicry can have a destabilising impact on species coexistence. The results not only contribute to our understanding of how mimicry is maintained in natural systems but also demonstrate how changes in relative abundance or population structure of one species could impact another species. Further insight into the population and community dynamics of insects is particularly important given the current population declines (Goulson 2019; Seibold et al 2019). References Bates, H. W. 1862. Contributions to the insect fauna of the Amazon Valley, Lepidoptera: Heliconidae. Trans. Linn. Soc. Lond. 23:495- 566. https://doi.org/10.1111/j.1096-3642.1860.tb00146.x Boutin, M., Costa, M., Fontaine, C., Perrard, A., Llaurens, V. 2022 Influence of sex-limited mimicry on extinction risk in Aculeata: a theoretical approach. bioRxiv, ver. 2 peer-reviewed and recommended by Peer Community in Ecology. https://doi.org/10.1101/2022.10.21.513153 Goulson, D. 2019. The insect apocalypse, and why it matters. Curr. Biol. 29: R967-R971. https://doi.org/10.1016/j.cub.2019.06.069 Müller, F. 1879. Ituna and Thyridia; a remarkable case of mimicry in butterflies. Trans. Roy. Entom. Roc. 1879:20-29. Seibold, S., Gossner, M. M., Simons, N. K., Blüthgen, N., Müller, J., Ambarlı, D., ... & Weisser, W. W. 2019. Arthropod decline in grasslands and forests is associated with landscape-level drivers. Nature, 574: 671-674. https://doi.org/10.1038/s41586-019-1684-3 | Influence of mimicry on extinction risk in Aculeata: a theoretical approach | Maxime Boutin, Manon Costa, Colin Fontaine, Adrien Perrard, Violaine Llaurens | <p style="text-align: justify;">Positive ecological interactions, such as mutualism, can play a role in community structure and species co-existence. A well-documented case of mutualistic interaction is Mullerian mimicry, the convergence of colour... | ![]() | Biodiversity, Coexistence, Eco-evolutionary dynamics, Evolutionary ecology, Facilitation & Mutualism | Amanda Franklin | 2022-10-25 19:11:55 | View | |
24 May 2022
![]() Controversy over the decline of arthropods: a matter of temporal baseline?François Duchenne, Emmanuelle Porcher, Jean-Baptiste Mihoub, Grégoire Loïs, Colin Fontaine https://doi.org/10.1101/2022.02.09.479422Don't jump to conclusions on arthropod abundance dynamics without appropriate dataRecommended by Tim CoulsonHumans are dramatically modifying many aspects of our planet via increasing concentrations of carbon dioxide in the atmosphere, patterns of land-use change, and unsustainable exploitation of the planet’s resources. These changes impact the abundance of species of wild organisms, with winners and losers. Identifying how different species and groups of species are influenced by anthropogenic activity in different biomes, continents, and habitats, has become a pressing scientific question with many publications reporting analyses of disparate data on species population sizes. Many conclusions are based on the linear analysis of rather short time series of organismal abundances. Duchenne F, Porcher E, Mihoub J-B, Loïs G, Fontaine C (2022) Controversy over the decline of arthropods: a matter of temporal baseline? bioRxiv, 2022.02.09.479422, ver. 3 peer-reviewed and recommended by Peer Community in Ecology. https://doi.org/10.1101/2022.02.09.479422 | Controversy over the decline of arthropods: a matter of temporal baseline? | François Duchenne, Emmanuelle Porcher, Jean-Baptiste Mihoub, Grégoire Loïs, Colin Fontaine | <p style="text-align: justify;">Recently, a number of studies have reported somewhat contradictory patterns of temporal trends in arthropod abundance, from decline to increase. Arthropods often exhibit non-monotonous variation in abundance over ti... | ![]() | Conservation biology | Tim Coulson | 2022-02-11 15:44:44 | View |
MANAGING BOARD
Julia Astegiano
Tim Coulson
Anna Eklof
Dominique Gravel
François Massol
Ben Phillips
Cyrille Violle