Direct submissions to PCI Ecology from bioRxiv.org are possible using the B2J service
Latest recommendations
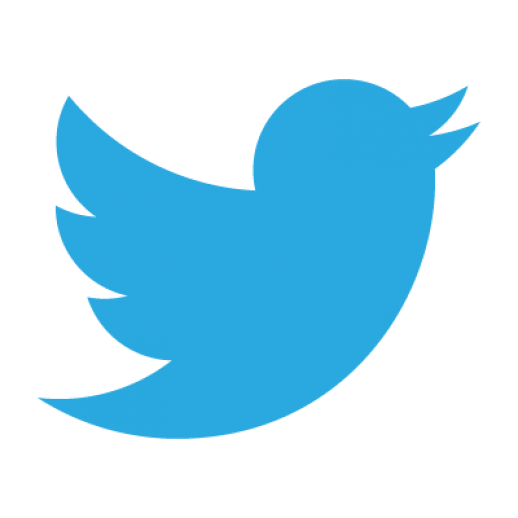
Id | Title * | Authors * | Abstract * | Picture * ▲ | Thematic fields * | Recommender | Reviewers | Submission date | |
---|---|---|---|---|---|---|---|---|---|
03 Apr 2020
![]() A macro-ecological approach to predators' functional responseMatthieu Barbier, Laurie Wojcik, Michel Loreau https://doi.org/10.1101/832220A meta-analysis to infer generic predator functional responseRecommended by Samir Simon Suweis based on reviews by Ludek Berec and gyorgy barabasSpecies interactions are classically derived from the law of mass action: the probability that, for example, a predation event occurs is proportional to the product of the density of the prey and predator species. In order to describe how predator and prey species populations grow, is then necessary to introduce functional response, describing the intake rate of a consumer as a function of food (e.g. prey) density. References [1] Volterra, V. (1928). Variations and Fluctuations of the Number of Individuals in Animal Species living together. ICES Journal of Marine Science, 3(1), 3–51. doi: 10.1093/icesjms/3.1.3 | A macro-ecological approach to predators' functional response | Matthieu Barbier, Laurie Wojcik, Michel Loreau | <p>Predation often deviates from the law of mass action: many micro- and meso-scale experiments have shown that consumption saturates with resource abundance, and decreases due to interference between consumers. But does this observation hold at m... | ![]() | Community ecology, Food webs, Meta-analyses, Theoretical ecology | Samir Simon Suweis | 2019-11-08 15:42:16 | View | |
12 Jan 2024
![]() Methods for tagging an ectoparasite, the salmon louse Lepeophtheirus salmonisAlexius Folk, Adele Mennerat https://doi.org/10.1101/2023.08.31.555695Marking invertebrates using RFID tagsRecommended by Nicolas SchtickzelleGuiding and monitoring the efficiency of conservation efforts needs robust scientific background information, of which one key element is estimating wildlife abundance and its spatial and temporal variation. As raw counts are by nature incomplete counts of a population, correcting for detectability is required (Clobert, 1995; Turlure et al., 2018). This can be done with Capture-Mark-Recapture protocols (Iijima, 2020). Techniques for marking individuals are diverse, e.g. writing on butterfly wings, banding birds, or using natural specific patterns in the individual’s body such as leopard fur or whale tail. Advancement in technology opens new opportunities for developing marking techniques, including strategies to limit mark identification errors (Burchill & Pavlic, 2019), and for using active marks that can transmit data remotely or be read automatically. The details of such methodological developments frequently remain unpublished, the method being briefly described in studies that use it. For a few years, there has been however a renewed interest in proper publishing of methods for ecology and evolution. This study by Folk & Mennerat (2023) fits in this context, offering a nice example of detailed description and testing of a method to mark salmon ectoparasites using RFID tags. Such tags are extremely small, yet easy to use, even with automatic recording procedure. The study provides a very good basis protocol that should help researchers working for small species, in particular invertebrates. The study is complemented by a video illustrating the placement of the tag so the reader who would like to replicate the procedure can get a very precise idea of it. References Burchill, A. T., & Pavlic, T. P. (2019). Dude, where’s my mark? Creating robust animal identification schemes informed by communication theory. Animal Behaviour, 154, 203–208. https://doi.org/10.1016/j.anbehav.2019.05.013 Clobert, J. (1995). Capture-recapture and evolutionary ecology: A difficult wedding ? Journal of Applied Statistics, 22(5–6), 989–1008. Folk, A., & Mennerat, A. (2023). Methods for tagging an ectoparasite, the salmon louse Lepeophtheirus salmonis (p. 2023.08.31.555695). bioRxiv, ver. 2 peer-reviewed and recommended by Peer Community in Ecology. https://doi.org/10.1101/2023.08.31.555695 Iijima, H. (2020). A Review of Wildlife Abundance Estimation Models: Comparison of Models for Correct Application. Mammal Study, 45(3), 177–188. https://doi.org/10.3106/ms2019-0082 Turlure, C., Pe’er, G., Baguette, M., & Schtickzelle, N. (2018). A simplified mark–release–recapture protocol to improve the cost effectiveness of repeated population size quantification. Methods in Ecology and Evolution, 9(3), 645–656. https://doi.org/10.1111/2041-210X.12900 | Methods for tagging an ectoparasite, the salmon louse *Lepeophtheirus salmonis* | Alexius Folk, Adele Mennerat | <p style="text-align: justify;">Monitoring individuals within populations is a cornerstone in evolutionary ecology, yet individual tracking of invertebrates and particularly parasitic organisms remains rare. To address this gap, we describe here a... | ![]() | Dispersal & Migration, Evolutionary ecology, Host-parasite interactions, Marine ecology, Parasitology, Terrestrial ecology, Zoology | Nicolas Schtickzelle | 2023-09-04 15:25:08 | View | |
03 Mar 2022
![]() Artificial reefs geographical location matters more than its age and depth for sessile invertebrate colonization in the Gulf of Lion (NorthWestern Mediterranean Sea)sylvain blouet, Katell Guizien, lorenzo Bramanti https://doi.org/10.1101/2021.10.08.463669A longer-term view on benthic communities on artificial reefs: it’s all about locationRecommended by James Davis Reimer based on reviews by 2 anonymous reviewersIn this study by Blouet, Bramanti, and Guizen (2022), the authors aim to tackle a long-standing data gap regarding research on marine benthic communities found on artificial reefs. The study is well thought out, and should serve as an important reference on this topic going forward. Blouet S, Bramanti L, Guizien K (2022) Artificial reefs geographical location matters more than shape, age and depth for sessile invertebrate colonization in the Gulf of Lion (NorthWestern Mediterranean Sea). bioRxiv, 2021.10.08.463669, ver. 4 peer-reviewed and recommended by Peer Community in Ecology. https://doi.org/10.1101/2021.10.08.463669 | Artificial reefs geographical location matters more than its age and depth for sessile invertebrate colonization in the Gulf of Lion (NorthWestern Mediterranean Sea) | sylvain blouet, Katell Guizien, lorenzo Bramanti | <p>Artificial reefs (ARs) have been used to support fishing activities. Sessile invertebrates are essential components of trophic networks within ARs, supporting fish productivity. However, colonization by sessile invertebrates is possible only af... | ![]() | Biodiversity, Biogeography, Colonization, Ecological successions, Life history, Marine ecology | James Davis Reimer | 2021-10-11 10:21:36 | View | |
11 Mar 2024
Sex differences in the relationship between maternal and neonate cortisol in a free-ranging large mammalAmin, B., Fishman, R., Quinn, M., Matas, D., Palme, R., Koren, L., Ciuti, S. https://doi.org/10.1101/2023.05.04.538920Stress and stress hormones’ transmission from mothers to offspringRecommended by Matthieu PaquetIndividuals can respond to environmental changes that they undergo directly (within-generation plasticity) but also through transgenerational plasticity, providing lasting effects that are transmitted to the next generations (Donelson et al. 2012; Munday et al. 2013; Kuijper & Hoyle 2015; Auge et al. 2017, Tariel et al. 2020). These parental effects can affect offspring via various mechanisms, notably via maternal transmission of hormones to the eggs or growing embryos (Mousseau & Fox 1998). While the effects of environmental quality may simply carry-over to the next generation (e.g., females in stressful environments give birth to offspring in poorer condition), parental effects may also be a mechanism that adjusts offspring phenotype in response to environmental variation and predictability, and thereby match offspring's phenotype to future environmental conditions (Gluckman et al. 2005; Marshall & Uller 2007; Dey et al. 2016; Yin et al. 2019), for example by preparing their offspring to an expected stressful environment. When females experience stress during gestation or egg formation, elevations in glucocorticoids (GC) are expected to affect offspring phenotype in many ways, from the offspring's own GC levels, to their growth and survival (Sheriff et al. 2017). This is a well established idea, but how strong is the evidence for this? A meta-analysis on birds found no clear effect of corticosterone manipulation on offspring traits (38 studies on 9 bird species for corticosterone manipulation; Podmokła et al. 2018). Another meta-analysis including 14 vertebrate species found no clear effect of prenatal stress on offspring GC (Thayer et al. 2018). Finally, a meta-analysis on wild vertebrates (23 species) found no clear effect of GC-mediated maternal effects on offspring traits (MacLeod et al. 2021). As often when facing such inconclusive results, context dependence has been suggested as one potential reason for such inconsistencies, for exemple sex specific effects (Groothuis et al. 2019, 2020). However, sex specific measures on offspring are scarce (Podmokła et al. 2018). Moreover, the literature available is still limited to a few, mostly “model” species. With their study, Amin et al. (2024) show the way to improve our understanding on GC transmission from mother to offspring and its effects in several aspects. First they used innovative non-invasive methods (which could broaden the range of species available to study) by quantifying cortisol metabolites from faecal samples collected from pregnant females, as proxy for maternal GC level, and relating it to GC levels from hairs of their neonate offspring. Second they used a free ranging large mammal (taxa from which literature is missing): the fallow deer (Dama dama). Third, they provide sex specific measures of GC levels. And finally but importantly, they are exemplary in their transparency regarding 1) the exploratory nature of their study, 2) their statistical thinking and procedure, and 3) the study limitations (e.g., low sample size and high within individual variation of measurements). I hope this study will motivate more research (on the fallow deer, and on other species) to broaden and strengthen our understanding of sex specific effects of maternal stress and CG levels on offspring phenotype and fitness. References Amin, B., Fishman, R., Quinn, M., Matas, D., Palme, R., Koren, L., & Ciuti, S. (2024). Sex differences in the relationship between maternal and foetal glucocorticoids in a free-ranging large mammal. bioRxiv, ver. 4 peer-reviewed and recommended by Peer Community in Ecology. https://doi.org/10.1101/2023.05.04.538920 Auge, G.A., Leverett, L.D., Edwards, B.R. & Donohue, K. (2017). Adjusting phenotypes via within-and across-generational plasticity. New Phytologist, 216, 343–349. https://doi.org/10.1111/nph.14495 Dey, S., Proulx, S.R. & Teotonio, H. (2016). Adaptation to temporally fluctuating environments by the evolution of maternal effects. PLoS biology, 14, e1002388. https://doi.org/10.1371/journal.pbio.1002388 Donelson, J.M., Munday, P.L., McCormick, M.I. & Pitcher, C.R. (2012). Rapid transgenerational acclimation of a tropical reef fish to climate change. Nature Climate Change, 2, 30. https://doi.org/10.1038/nclimate1323 Gluckman, P.D., Hanson, M.A. & Spencer, H.G. (2005). Predictive adaptive responses and human evolution. Trends in ecology & evolution, 20, 527–533. https://doi.org/10.1016/j.tree.2005.08.001 Groothuis, Ton GG, Bin-Yan Hsu, Neeraj Kumar, and Barbara Tschirren. "Revisiting mechanisms and functions of prenatal hormone-mediated maternal effects using avian species as a model." Philosophical Transactions of the Royal Society B 374, no. 1770 (2019): 20180115. https://doi.org/10.1098/rstb.2018.0115 Groothuis, Ton GG, Neeraj Kumar, and Bin-Yan Hsu. "Explaining discrepancies in the study of maternal effects: the role of context and embryo." Current Opinion in Behavioral Sciences 36 (2020): 185-192. https://doi.org/10.1016/j.cobeha.2020.10.006 Kuijper, B. & Hoyle, R.B. (2015). When to rely on maternal effects and when on phenotypic plasticity? Evolution, 69, 950–968. https://doi.org/10.1111/evo.12635 MacLeod, Kirsty J., Geoffrey M. While, and Tobias Uller. "Viviparous mothers impose stronger glucocorticoid‐mediated maternal stress effects on their offspring than oviparous mothers." Ecology and Evolution 11, no. 23 (2021): 17238-17259. Marshall, D.J. & Uller, T. (2007). When is a maternal effect adaptive? Oikos, 116, 1957–1963. https://doi.org/10.1111/j.2007.0030-1299.16203.x Mousseau, T.A. & Fox, C.W. (1998). Maternal effects as adaptations. Oxford University Press. Munday, P.L., Warner, R.R., Monro, K., Pandolfi, J.M. & Marshall, D.J. (2013). Predicting evolutionary responses to climate change in the sea. Ecology Letters, 16, 1488–1500. https://doi.org/10.1111/ele.12185 Podmokła, Edyta, Szymon M. Drobniak, and Joanna Rutkowska. "Chicken or egg? Outcomes of experimental manipulations of maternally transmitted hormones depend on administration method–a meta‐analysis." Biological Reviews 93, no. 3 (2018): 1499-1517. https://doi.org/10.1111/brv.12406 Sheriff, M. J., Bell, A., Boonstra, R., Dantzer, B., Lavergne, S. G., McGhee, K. E., MacLeod, K. J., Winandy, L., Zimmer, C., & Love, O. P. (2017). Integrating ecological and evolutionary context in the study of maternal stress. Integrative and Comparative Biology, 57(3), 437–449. https://doi.org/10.1093/icb/icx105 Tariel, Juliette, Sandrine Plénet, and Émilien Luquet. "Transgenerational plasticity in the context of predator-prey interactions." Frontiers in Ecology and Evolution 8 (2020): 548660. https://doi.org/10.3389/fevo.2020.548660 Thayer, Zaneta M., Meredith A. Wilson, Andrew W. Kim, and Adrian V. Jaeggi. "Impact of prenatal stress on offspring glucocorticoid levels: A phylogenetic meta-analysis across 14 vertebrate species." Scientific Reports 8, no. 1 (2018): 4942. https://doi.org/10.1038/s41598-018-23169-w Yin, J., Zhou, M., Lin, Z., Li, Q.Q. & Zhang, Y.-Y. (2019). Transgenerational effects benefit offspring across diverse environments: a meta-analysis in plants and animals. Ecology letters, 22, 1976–1986. https://doi.org/10.1111/ele.13373 | Sex differences in the relationship between maternal and neonate cortisol in a free-ranging large mammal | Amin, B., Fishman, R., Quinn, M., Matas, D., Palme, R., Koren, L., Ciuti, S. | <p style="text-align: justify;">Maternal phenotypes can have long-term effects on offspring phenotypes. These maternal effects may begin during gestation, when maternal glucocorticoid (GC) levels may affect foetal GC levels, thereby having an orga... | Evolutionary ecology, Maternal effects, Ontogeny, Physiology, Zoology | Matthieu Paquet | 2023-06-05 09:06:56 | View | ||
16 Oct 2018
Impact of group management and transfer on individual sociality in Highland cattle (Bos Taurus)Sebastian Sosa, Marie Pelé, Elise Debergue, Cedric Kuntz, Blandine Keller, Florian Robic, Flora Siegwalt-Baudin, Camille Richer, Amandine Ramos, Cédric Sueur https://arxiv.org/abs/1805.11553v4How empirical sciences may improve livestock welfare and help their managementRecommended by Marie Charpentier based on reviews by Alecia CARTER and 1 anonymous reviewerUnderstanding how livestock management is a source of social stress and disturbances for cattle is an important question with potential applications for animal welfare programs and sustainable development. In their article, Sosa and colleagues [1] first propose to evaluate the effects of individual characteristics on dyadic social relationships and on the social dynamics of four groups of cattle. Using network analyses, the authors provide an interesting and complete picture of dyadic interactions among groupmates. Although shown elsewhere, the authors demonstrate that individuals that are close in age and close in rank form stronger dyadic associations than other pairs. Second, the authors take advantage of some transfers of animals between groups -for management purposes- to assess how these transfers affect the social dynamics of groupmates. Their central finding is that the identity of transferred animals is a key-point. In particular, removing offspring strongly destabilizes the social relationships of mothers while adding a bull into a group also profoundly impacts female-female social relationships, as social networks before and after transfer of these key-animals are completely different. In addition, individuals, especially the young ones, that are transferred without familiar conspecifics take more time to socialize with their new group members than individuals transferred with familiar groupmates, generating a potential source of stress. Interestingly, the authors end up their article with some thoughts on the implications of their findings for animal welfare and ethics. This study provides additional evidence that empirical science has a major role to play in providing recommendations regarding societal questions such as livestock management and animal wellbeing. References [1] Sosa, S., Pelé, M., Debergue, E., Kuntz, C., Keller, B., Robic, F., Siegwalt-Baudin, F., Richer, C., Ramos, A., & Sueur C. (2018). Impact of group management and transfer on individual sociality in Highland cattle (Bos Taurus). arXiv:1805.11553v4 [q-bio.PE] peer-reviewed and recommended by PCI Ecol. https://arxiv.org/abs/1805.11553v4 | Impact of group management and transfer on individual sociality in Highland cattle (Bos Taurus) | Sebastian Sosa, Marie Pelé, Elise Debergue, Cedric Kuntz, Blandine Keller, Florian Robic, Flora Siegwalt-Baudin, Camille Richer, Amandine Ramos, Cédric Sueur | The sociality of cattle facilitates the maintenance of herd cohesion and synchronisation, making these species the ideal choice for domestication as livestock for humans. However, livestock populations are not self-regulated, and farmers transfer ... | Behaviour & Ethology, Social structure | Marie Charpentier | 2018-05-30 14:05:39 | View | ||
15 Feb 2024
![]() Sources of confusion in global biodiversity trendsMaelys Boennec, Vasilis Dakos, Vincent Devictor https://doi.org/10.32942/X29W3HUnraveling the Complexity of Global Biodiversity Dynamics: Insights and ImperativesRecommended by Paulo BorgesBiodiversity loss is occurring at an alarming rate across terrestrial and marine ecosystems, driven by various processes that degrade habitats and threaten species with extinction. Despite the urgency of this issue, empirical studies present a mixed picture, with some indicating declining trends while others show more complex patterns. In a recent effort to better understand global biodiversity dynamics, Boennec et al. (2024) conducted a comprehensive literature review examining temporal trends in biodiversity. Their analysis reveals that reviews and meta-analyses, coupled with the use of global indicators, tend to report declining trends more frequently. Additionally, the study underscores a critical gap in research: the scarcity of investigations into the combined impact of multiple pressures on biodiversity at a global scale. This lack of understanding complicates efforts to identify the root causes of biodiversity changes and develop effective conservation strategies. This study serves as a crucial reminder of the pressing need for long-term biodiversity monitoring and large-scale conservation studies. By filling these gaps in knowledge, researchers can provide policymakers and conservation practitioners with the insights necessary to mitigate biodiversity loss and safeguard ecosystems for future generations. References Boennec, M., Dakos, V. & Devictor, V. (2023). Sources of confusion in global biodiversity trend. bioRxiv, ver. 4 peer-reviewed and recommended by Peer Community in Ecology. https://doi.org/10.32942/X29W3H
| Sources of confusion in global biodiversity trends | Maelys Boennec, Vasilis Dakos, Vincent Devictor | <p>Populations and ecological communities are changing worldwide, and empirical studies exhibit a mixture of either declining or mixed trends. Confusion in global biodiversity trends thus remains while assessing such changes is of major social, po... | ![]() | Biodiversity, Conservation biology, Meta-analyses | Paulo Borges | 2023-09-20 11:10:25 | View | |
31 Oct 2022
![]() Ten simple rules for working with high resolution remote sensing dataAdam L. Mahood, Maxwell Benjamin Joseph, Anna Spiers, Michael J. Koontz, Nayani Ilangakoon, Kylen Solvik, Nathan Quarderer, Joe McGlinchy, Victoria Scholl, Lise St. Denis, Chelsea Nagy, Anna Braswell, Matthew W. Rossi, Lauren Herwehe, Leah wasser, Megan Elizabeth Cattau, Virginia Iglesias, Fangfang Yao, Stefan Leyk, Jennifer Balch https://doi.org/10.31219/osf.io/kehqzPreventing misuse of high-resolution remote sensing dataRecommended by Eric GobervilleTo observe, characterise, identify, understand, predict... This is the approach that researchers follow every day. This sequence is tirelessly repeated as the biological model, the targeted ecosystem and/or the experimental, environmental or modelling conditions change. This way of proceeding is essential in a world of rapid change in response to the frenetic pace of intensifying pressures and forcings that impact ecosystems. To better understand our Earth and the dynamics of its components, to map ecosystems and diversity patterns, and to identify changes, humanity had to demonstrate inventiveness and defy gravity. Gustave Hermite and Georges Besançon were the first to launch aloft balloons equipped with radio transmitters, making possible the transmission of meteorological data to observers in real time [1]. The development of aviation in the middle of the 20th century constituted a real leap forward for the frequent acquisition of aerial observations, leading to a significant improvement in weather forecasting models. The need for systematic collection of data as holistic as possible – an essential component for the observation of complex biological systems - has resulted in pushing the limits of technological prowess. The conquest of space and the concurrent development of satellite observations has largely contributed to the collection of a considerable mass of data, placing our Earth under the "macroscope" - a concept introduced to ecology in the early 1970s by Howard T. Odum (see [2]), and therefore allowing researchers to move towards a better understanding of ecological systems, deterministic and stochastic patterns … with the ultimate goal of improving management actions [2,3]. Satellite observations have been carried out for nearly five decades now [3] and have greatly contributed to a better qualitative and quantitative understanding of the functioning of our planet, its diversity, its climate... and to a better anticipation of possible future changes (e.g., [4-7]). This access to rich and complex sources of information, for which both spatial and temporal resolutions are increasingly fine, results in the implementation of increasingly complex computation-based analyses, in order to meet the need for a better understanding of ecological mechanisms and processes, and their possible changes. Steven Levitt stated that "Data is one of the most powerful mechanisms for telling stories". This is so true … Data should not be used as a guide to thinking and a critical judgment at each stage of the data exploitation process should not be neglected. This is what Mahood et al. [8] rightly remind us in their article "Ten simple rules for working with high-resolution remote sensing data" in which they provide the fundamentals to consider when working with data of this nature, a still underutilized resource in several topics, such as conservation biology [3]. In this unconventional article, presented in a pedagogical way, the authors remind different generations of readers how satellite data should be handled and processed. The authors aim to make the readers aware of the most frequent pitfalls encouraging them to use data adapted to their original question, the most suitable tools/methods/procedures, to avoid methodological overkill, and to ensure both ethical use of data and transparency in the research process. While access to high-resolution data is increasingly easy thanks to the implementation of dedicated platforms [4], and because of the development of easy-to-use processing software and pipelines, it is important to take the time to recall some of the essential rules and guidelines for managing them, from new users with little or no experience who will find in this article the recommendations, resources and advice necessary to start exploiting remote sensing data, to more experienced researchers. References [1] Jeannet P, Philipona R, and Richner H (2016). 8 Swiss upper-air balloon soundings since 1902. In: Willemse S, Furger M (2016) From weather observations to atmospheric and climate sciences in Switzerland: Celebrating 100 years of the Swiss Society for Meteorology. vdf Hochschulverlag AG. [2] Odum HT (2007) Environment, Power, and Society for the Twenty-First Century: The Hierarchy of Energy. Columbia University Press. [3] Boyle SA, Kennedy CM, Torres J, Colman K, Pérez-Estigarribia PE, Sancha NU de la (2014) High-Resolution Satellite Imagery Is an Important yet Underutilized Resource in Conservation Biology. PLOS ONE, 9, e86908. https://doi.org/10.1371/journal.pone.0086908 [4] Le Traon P-Y, Antoine D, Bentamy A, Bonekamp H, Breivik LA, Chapron B, Corlett G, Dibarboure G, DiGiacomo P, Donlon C, Faugère Y, Font J, Girard-Ardhuin F, Gohin F, Johannessen JA, Kamachi M, Lagerloef G, Lambin J, Larnicol G, Le Borgne P, Leuliette E, Lindstrom E, Martin MJ, Maturi E, Miller L, Mingsen L, Morrow R, Reul N, Rio MH, Roquet H, Santoleri R, Wilkin J (2015) Use of satellite observations for operational oceanography: recent achievements and future prospects. Journal of Operational Oceanography, 8, s12–s27. https://doi.org/10.1080/1755876X.2015.1022050 [5] Turner W, Rondinini C, Pettorelli N, Mora B, Leidner AK, Szantoi Z, Buchanan G, Dech S, Dwyer J, Herold M, Koh LP, Leimgruber P, Taubenboeck H, Wegmann M, Wikelski M, Woodcock C (2015) Free and open-access satellite data are key to biodiversity conservation. Biological Conservation, 182, 173–176. https://doi.org/10.1016/j.biocon.2014.11.048 [6] Melet A, Teatini P, Le Cozannet G, Jamet C, Conversi A, Benveniste J, Almar R (2020) Earth Observations for Monitoring Marine Coastal Hazards and Their Drivers. Surveys in Geophysics, 41, 1489–1534. https://doi.org/10.1007/s10712-020-09594-5 [7] Zhao Q, Yu L, Du Z, Peng D, Hao P, Zhang Y, Gong P (2022) An Overview of the Applications of Earth Observation Satellite Data: Impacts and Future Trends. Remote Sensing, 14, 1863. https://doi.org/10.3390/rs14081863 [8] Mahood AL, Joseph MB, Spiers A, Koontz MJ, Ilangakoon N, Solvik K, Quarderer N, McGlinchy J, Scholl V, Denis LS, Nagy C, Braswell A, Rossi MW, Herwehe L, Wasser L, Cattau ME, Iglesias V, Yao F, Leyk S, Balch J (2021) Ten simple rules for working with high resolution remote sensing data. OSFpreprints, ver. 6 peer-reviewed and recommended by Peer Community in Ecology. https://doi.org/10.31219/osf.io/kehqz | Ten simple rules for working with high resolution remote sensing data | Adam L. Mahood, Maxwell Benjamin Joseph, Anna Spiers, Michael J. Koontz, Nayani Ilangakoon, Kylen Solvik, Nathan Quarderer, Joe McGlinchy, Victoria Scholl, Lise St. Denis, Chelsea Nagy, Anna Braswell, Matthew W. Rossi, Lauren Herwehe, Leah wasser,... | <p>Researchers in Earth and environmental science can extract incredible value from high-resolution (sub-meter, sub-hourly or hyper-spectral) remote sensing data, but these data can be difficult to use. Correct, appropriate and competent use of su... | ![]() | Biogeography, Landscape ecology, Macroecology, Spatial ecology, Metacommunities & Metapopulations, Terrestrial ecology | Eric Goberville | 2021-10-19 21:41:22 | View | |
10 Jan 2019
![]() Inferring macro-ecological patterns from local species' occurrencesAnna Tovo, Marco Formentin, Samir Suweis, Samuele Stivanello, Sandro Azaele, Amos Maritan https://doi.org/10.1101/387456Upscaling the neighborhood: how to get species diversity, abundance and range distributions from local presence/absence dataRecommended by Matthieu BarbierHow do you estimate the biodiversity of a whole community, or the distribution of abundances and ranges of its species, from presence/absence data in scattered samples? ADDITIONAL COMMENTS 1) To explain the novelty of the authors' contribution, it is useful to look at competing techniques. 2) The main condition for all such approaches to work is well-mixedness: each sample should be sufficiently like a lot drawn from the same skewed lottery. As long as that condition applies, finding the best approach is a theoretical matter of probabilities and combinatorics that may, in time, be given a definite answer. 3) One may ask: why the Negative Binomial as a Species Abundance Distribution? References [1] Fisher, R. A., Corbet, A. S., & Williams, C. B. (1943). The relation between the number of species and the number of individuals in a random sample of an animal population. The Journal of Animal Ecology, 42-58. doi: 10.2307/1411 | Inferring macro-ecological patterns from local species' occurrences | Anna Tovo, Marco Formentin, Samir Suweis, Samuele Stivanello, Sandro Azaele, Amos Maritan | <p>Biodiversity provides support for life, vital provisions, regulating services and has positive cultural impacts. It is therefore important to have accurate methods to measure biodiversity, in order to safeguard it when we discover it to be thre... | ![]() | Macroecology, Species distributions, Statistical ecology, Theoretical ecology | Matthieu Barbier | 2018-08-09 16:44:09 | View | |
27 Apr 2021
![]() Joint species distributions reveal the combined effects of host plants, abiotic factors and species competition as drivers of species abundances in fruit fliesBenoit Facon, Abir Hafsi, Maud Charlery de la Masselière, Stéphane Robin, François Massol, Maxime Dubart, Julien Chiquet, Enric Frago, Frédéric Chiroleu, Pierre-François Duyck & Virginie Ravigné https://doi.org/10.1101/2020.12.07.414326Understanding the interplay between host-specificity, environmental conditions and competition through the sound application of Joint Species Distribution ModelsRecommended by Joaquín HortalUnderstanding why and how species coexist in local communities is one of the central questions in ecology. There is general agreement that species distribution and coexistence are determined by a number of key mechanisms, including the environmental requirements of species, dispersal, evolutionary constraints, resource availability and selection, metapopulation dynamics, and biotic interactions (e.g. Soberón & Nakamura 2009; Colwell & Rangel 2009; Ricklefs 2015). These factors are however intricately intertwined in a scale-structured fashion (Hortal et al. 2010; D’Amen et al. 2017), making it particularly difficult to tease apart the effects of each one of them. This could be addressed by the novel field of Joint Species Distribution Modelling (JSDM; Okasvainen & Abrego 2020), as it allows assessing the effects of several sets of factors and the co-occurrence and/or covariation in abundances of potentially interacting species at the same time (Pollock et al. 2014; Ovaskainen et al. 2016; Dormann et al. 2018). However, the development of JSDM has been hampered by the general lack of good-quality detailed data on species co-occurrences and abundances (see Hortal et al. 2015). Facon et al. (2021) use a particularly large compilation of field surveys to study the abundance and co-occurrence of Tephritidae fruit flies in c. 400 orchards, gardens and natural areas throughout the island of Réunion. Further, they combine such information with lab data on their host-selection fundamental niche (i.e. in the absence of competitors), codifying traits of female choice and larval performances in 21 host species. They use Poisson Log-Normal models, a type of mixed model that allows one to jointly model the random effects associated with all species, and retrieve the covariations in abundance that are not explained by environmental conditions or differences in sampling effort. Then, they use a series of models to evaluate the effects on these matrices of ecological covariates (date, elevation, habitat, climate and host plant), species interactions (by comparing with a constrained residual variance-covariance matrix) and the species’ host-selection fundamental niches (through separate models for each fly species). The eight Tephritidae species inhabiting Réunion include both generalists and specialists in Solanaceae and Cucurbitaceae with a known history of interspecific competition. Facon et al. (2021) use a comprehensive JSDM approach to assess the effects of different factors separately and altogether. This allows them to identify large effects of plant hosts and the fundamental host-selection niche on species co-occurrence, but also to show that ecological covariates and weak –though not negligible– species interactions are necessary to account for all residual variance in the matrix of joint species abundances per site. Further, they also find evidence that the fitness per host measured in the lab has a strong influence on the abundances in each host plant in the field for specialist species, but not for generalists. Indeed, the stronger effects of competitive exclusion were found in pairs of Cucurbitaceae specialist species. However, these analyses fail to provide solid grounds to assess why generalists are rarely found in Cucurbitaceae and Solanaceae. Although they argue that this may be due to Connell’s (1980) ghost of competition past (past competition that led to current niche differentiation), further data on the evolutionary history of these fruit flies is needed to assess this hypothesis. Finding evidence for the effects of competitive interactions on species’ occurrences and spatial distributions is often difficult, perhaps because these effects occur over longer time scales than the ones usually studied by ecologists (Yackulic 2017). The work by Facon and colleagues shows that weak effects of competition can be detected also at the short ecological timescales that determine coexistence in local communities, under the virtuous combination of good-quality data and sound analytical designs that account for several aspects of species’ niches, their biotopes and their joint population responses. This adds a new dimension to the application of Hutchinson’s (1978) niche framework to understand the spatial dynamics of species and communities (see also Colwell & Rangel 2009), although further advances to incorporate dispersal-driven metacommunity dynamics (see, e.g., Ovaskainen et al. 2016; Leibold et al. 2017) are certainly needed. Nonetheless, this work shows the potential value of in-depth analyses of species coexistence based on combining good-quality field data with well-thought out JSDM applications. If many studies like this are conducted, it is likely that the uprising field of Joint Species Distribution Modelling will improve our understanding of the hierarchical relationships between the different factors affecting species coexistence in ecological communities in the near future.
References Colwell RK, Rangel TF (2009) Hutchinson’s duality: The once and future niche. Proceedings of the National Academy of Sciences, 106, 19651–19658. https://doi.org/10.1073/pnas.0901650106 Connell JH (1980) Diversity and the Coevolution of Competitors, or the Ghost of Competition Past. Oikos, 35, 131–138. https://doi.org/10.2307/3544421 D’Amen M, Rahbek C, Zimmermann NE, Guisan A (2017) Spatial predictions at the community level: from current approaches to future frameworks. Biological Reviews, 92, 169–187. https://doi.org/10.1111/brv.12222 Dormann CF, Bobrowski M, Dehling DM, Harris DJ, Hartig F, Lischke H, Moretti MD, Pagel J, Pinkert S, Schleuning M, Schmidt SI, Sheppard CS, Steinbauer MJ, Zeuss D, Kraan C (2018) Biotic interactions in species distribution modelling: 10 questions to guide interpretation and avoid false conclusions. Global Ecology and Biogeography, 27, 1004–1016. https://doi.org/10.1111/geb.12759 Facon B, Hafsi A, Masselière MC de la, Robin S, Massol F, Dubart M, Chiquet J, Frago E, Chiroleu F, Duyck P-F, Ravigné V (2021) Joint species distributions reveal the combined effects of host plants, abiotic factors and species competition as drivers of community structure in fruit flies. bioRxiv, 2020.12.07.414326. ver. 4 peer-reviewed and recommended by Peer community in Ecology. https://doi.org/10.1101/2020.12.07.414326 Hortal J, de Bello F, Diniz-Filho JAF, Lewinsohn TM, Lobo JM, Ladle RJ (2015) Seven Shortfalls that Beset Large-Scale Knowledge of Biodiversity. Annual Review of Ecology, Evolution, and Systematics, 46, 523–549. https://doi.org/10.1146/annurev-ecolsys-112414-054400 Hortal J, Roura‐Pascual N, Sanders NJ, Rahbek C (2010) Understanding (insect) species distributions across spatial scales. Ecography, 33, 51–53. https://doi.org/10.1111/j.1600-0587.2009.06428.x Hutchinson, G.E. (1978) An introduction to population biology. Yale University Press, New Haven, CT. Leibold MA, Chase JM, Ernest SKM (2017) Community assembly and the functioning of ecosystems: how metacommunity processes alter ecosystems attributes. Ecology, 98, 909–919. https://doi.org/10.1002/ecy.1697 Ovaskainen O, Abrego N (2020) Joint Species Distribution Modelling: With Applications in R. Cambridge University Press, Cambridge. https://doi.org/10.1017/9781108591720 Ovaskainen O, Roy DB, Fox R, Anderson BJ (2016) Uncovering hidden spatial structure in species communities with spatially explicit joint species distribution models. Methods in Ecology and Evolution, 7, 428–436. https://doi.org/10.1111/2041-210X.12502 Pollock LJ, Tingley R, Morris WK, Golding N, O’Hara RB, Parris KM, Vesk PA, McCarthy MA (2014) Understanding co-occurrence by modelling species simultaneously with a Joint Species Distribution Model (JSDM). Methods in Ecology and Evolution, 5, 397–406. https://doi.org/10.1111/2041-210X.12180 Ricklefs RE (2015) Intrinsic dynamics of the regional community. Ecology Letters, 18, 497–503. https://doi.org/10.1111/ele.12431 Soberón J, Nakamura M (2009) Niches and distributional areas: Concepts, methods, and assumptions. Proceedings of the National Academy of Sciences, 106, 19644–19650. https://doi.org/10.1073/pnas.0901637106 Yackulic CB (2017) Competitive exclusion over broad spatial extents is a slow process: evidence and implications for species distribution modeling. Ecography, 40, 305–313. https://doi.org/10.1111/ecog.02836 | Joint species distributions reveal the combined effects of host plants, abiotic factors and species competition as drivers of species abundances in fruit flies | Benoit Facon, Abir Hafsi, Maud Charlery de la Masselière, Stéphane Robin, François Massol, Maxime Dubart, Julien Chiquet, Enric Frago, Frédéric Chiroleu, Pierre-François Duyck & Virginie Ravigné | <p style="text-align: justify;">The relative importance of ecological factors and species interactions for phytophagous insect species distributions has long been a controversial issue. Using field abundances of eight sympatric Tephritid fruit fli... | ![]() | Biodiversity, Coexistence, Community ecology, Competition, Herbivory, Interaction networks, Species distributions | Joaquín Hortal | Carsten Dormann, Joaquín Calatayud | 2020-12-08 06:44:25 | View |
14 Dec 2022
The contrasted impacts of grasshoppers on soil microbial activities in function of primary production and herbivore dietSébastien Ibanez, Arnaud Foulquier, Charles Brun, Marie-Pascale Colace, Gabin Piton, Lionel Bernard, Christiane Gallet, Jean-Christophe Clément https://doi.org/10.1101/2022.07.04.497718Complex interactions between ecosystem productivity and herbivore diets lead to non-predicted effects on nutrient cyclingRecommended by Sébastien Barot based on reviews by Manuel Blouin and Tord Ranheim SveenThe authors present a study typical of the field of belowground-aboveground interactions [1]. This framework has been extremely fruitful since the beginning of 2000s [2]. It has also contributed to bridge the gap between soil ecology and the rest of ecology [3]. The study also pertains to the rich field on the impacts of herbivores on soil functioning [4]. The study more precisely tested during two years the effect on nutrient cycling of the interaction between the type of grassland (along a gradient of biomass productivity) and the diet of the community of insect herbivores (5 treatments manipulating the grasshopper community on 1 m2 plots, with a gradient from no grasshopper to grasshoppers either specialized on forbs or grasses). What seems extremely interesting is that the study is based on a rigorous hypothesis-testing approach. They compare the predictions of two frameworks: (1) The “productivity model” predicts that in productive ecosystems herbivores consume a high percentage of the net primary production thus accelerating nutrient cycling. (2) The “diet model” distinguishes herbivores consuming exploitative plants from those eating conservative plants. The former (later) type of herbivores favours conservative (exploitative) plants therefore decelerating (accelerating) nutrient cycling. Interestingly, the two frameworks have similar predictions (and symmetrically opposite predictions) in two cases out of four combinations between ecosystem productivities and types of diet (see Table 1). An other merit of the study is to combine in a rather comprehensive way all the necessary measurements to test these frameworks in combination: grasshopper diet, soil properties, characteristics of the soil microbial community, plant traits, vegetation survey and plant biomass. The results were in contradiction with the ‘‘diet model’’: microbial properties and nitrogen cycling did not depend on grasshopper diet. The productivity of the grasslands did impact nutrient cycling but not in the direction predicted by the “productivity model”: productive grasslands hosted exploitative plants that depleted N resources in the soil and microbes producing few extracellular enzymes, which led to a lower potential N mineralization and a deceleration of nutrient cycling. Because, the authors stuck to their original hypotheses (that were not confirmed), they were able to discuss in a very relevant way their results and to propose some interpretations, at least partially based on the time scales involved by the productivity and diet models. Beyond all the merits of this article, I think that two issues remain largely open in relation with the dynamics of the studied systems, and would deserve future research efforts. First, on the ‘‘short’’ term (up to several decades), can we predict how the communities of plants, soil microbes, and herbivores interact to drive the dynamics of the ecosystems? Second, at the evolutionary time scale, can we understand and predict the interactions between the evolution of plant, microbe and herbivore strategies and the consequences for the functioning of the grasslands? The two issues are difficult because of the multiple feedbacks involved. One way to go further would be to complement the empirical approach with models along existing research avenues [5, 6]. References [1] Ibanez S, Foulquier A, Brun C, Colace M-P, Piton G, Bernard L, Gallet C, Clément J-C (2022) The contrasted impacts of grasshoppers on soil microbial activities in function of primary production and herbivore diet. bioRxiv, 2022.07.04.497718, ver. 2 peer-reviewed and recommended by Peer Community in Ecology. https://doi.org/10.1101/2022.07.04.497718 [2] Hooper, D. U., Bignell, D. E., Brown, V. K., Brussaard, L., Dangerfield, J. M., Wall, D. H., Wardle, D. A., Coleman, D. C., Giller, K. E., Lavelle, P., Van der Putten, W. H., De Ruiter, P. C., et al. 2000. Interactions between aboveground and belowground biodiversity in terretrial ecosystems: patterns, mechanisms, and feedbacks. BioScience, 50, 1049-1061. https://doi.org/10.1641/0006-3568(2000)050[1049:IBAABB]2.0.CO;2 [3] Barot, S., Blouin, M., Fontaine, S., Jouquet, P., Lata, J.-C., and Mathieu, J. 2007. A tale of four stories: soil ecology, theory, evolution and the publication system. PLoS ONE, 2, e1248. https://doi.org/10.1371/journal.pone.0001248 [4] Bardgett, R. D., and Wardle, D. A. 2003. Herbivore-mediated linkages between aboveground and belowground communities. Ecology, 84, 2258-2268. https://doi.org/10.1890/02-0274 [5] Barot, S., Bornhofen, S., Loeuille, N., Perveen, N., Shahzad, T., and Fontaine, S. 2014. Nutrient enrichment and local competition influence the evolution of plant mineralization strategy, a modelling approach. J. Ecol., 102, 357-366. https://doi.org/10.1111/1365-2745.12200 [6] Schweitzer, J. A., Juric, I., van de Voorde, T. F. J., Clay, K., van der Putten, W. H., Bailey, J. K., and Fox, C. 2014. Are there evolutionary consequences of plant-soil feedbacks along soil gradients? Func. Ecol., 28, 55-64. https://doi.org/10.1111/1365-2435.12201
| The contrasted impacts of grasshoppers on soil microbial activities in function of primary production and herbivore diet | Sébastien Ibanez, Arnaud Foulquier, Charles Brun, Marie-Pascale Colace, Gabin Piton, Lionel Bernard, Christiane Gallet, Jean-Christophe Clément | <p style="text-align: justify;">Herbivory can have contrasted impacts on soil microbes and nutrient cycling, which has stimulated the development of conceptual frameworks exploring the links between below- and aboveground processes. The "productiv... | Ecosystem functioning, Herbivory, Soil ecology, Terrestrial ecology | Sébastien Barot | 2022-07-14 09:06:13 | View |
MANAGING BOARD
Julia Astegiano
Tim Coulson
Anna Eklof
Dominique Gravel
François Massol
Ben Phillips
Cyrille Violle