Direct submissions to PCI Ecology from bioRxiv.org are possible using the B2J service
Latest recommendations
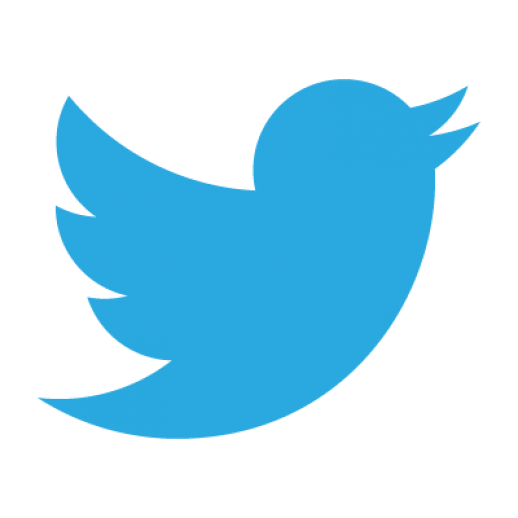
Id | Title * | Authors * | Abstract * | Picture * | Thematic fields * | Recommender | Reviewers▲ | Submission date | |
---|---|---|---|---|---|---|---|---|---|
11 Aug 2023
![]() Implementing Code Review in the Scientific Workflow: Insights from Ecology and Evolutionary BiologyEdward Ivimey-Cook, Joel Pick, Kevin Bairos-Novak, Antica Culina, Elliot Gould, Matthew Grainger, Benjamin Marshall, David Moreau, Matthieu Paquet, Raphaël Royauté, Alfredo Sanchez-Tojar, Inês Silva, Saras Windecker https://doi.org/10.32942/X2CG64A handy “How to” review code for ecologists and evolutionary biologistsRecommended by Corina LoganIvimey Cook et al. (2023) provide a concise and useful “How to” review code for researchers in the fields of ecology and evolutionary biology, where the systematic review of code is not yet standard practice during the peer review of articles. Consequently, this article is full of tips for authors on how to make their code easier to review. This handy article applies not only to ecology and evolutionary biology, but to many fields that are learning how to make code more reproducible and shareable. Taking this step toward transparency is key to improving research rigor (Brito et al. 2020) and is a necessary step in helping make research trustable by the public (Rosman et al. 2022). References Brito, J. J., Li, J., Moore, J. H., Greene, C. S., Nogoy, N. A., Garmire, L. X., & Mangul, S. (2020). Recommendations to enhance rigor and reproducibility in biomedical research. GigaScience, 9(6), giaa056. https://doi.org/10.1093/gigascience/giaa056 Ivimey-Cook, E. R., Pick, J. L., Bairos-Novak, K., Culina, A., Gould, E., Grainger, M., Marshall, B., Moreau, D., Paquet, M., Royauté, R., Sanchez-Tojar, A., Silva, I., Windecker, S. (2023). Implementing Code Review in the Scientific Workflow: Insights from Ecology and Evolutionary Biology. EcoEvoRxiv, ver 5 peer-reviewed and recommended by Peer Community In Ecology. https://doi.org/10.32942/X2CG64 Rosman, T., Bosnjak, M., Silber, H., Koßmann, J., & Heycke, T. (2022). Open science and public trust in science: Results from two studies. Public Understanding of Science, 31(8), 1046-1062. https://doi.org/10.1177/09636625221100686 | Implementing Code Review in the Scientific Workflow: Insights from Ecology and Evolutionary Biology | Edward Ivimey-Cook, Joel Pick, Kevin Bairos-Novak, Antica Culina, Elliot Gould, Matthew Grainger, Benjamin Marshall, David Moreau, Matthieu Paquet, Raphaël Royauté, Alfredo Sanchez-Tojar, Inês Silva, Saras Windecker | <p>Code review increases reliability and improves reproducibility of research. As such, code review is an inevitable step in software development and is common in fields such as computer science. However, despite its importance, code review is not... | ![]() | Meta-analyses, Statistical ecology | Corina Logan | 2023-05-19 15:54:01 | View | |
11 Mar 2024
Sex differences in the relationship between maternal and neonate cortisol in a free-ranging large mammalAmin, B., Fishman, R., Quinn, M., Matas, D., Palme, R., Koren, L., Ciuti, S. https://doi.org/10.1101/2023.05.04.538920Stress and stress hormones’ transmission from mothers to offspringRecommended by Matthieu PaquetIndividuals can respond to environmental changes that they undergo directly (within-generation plasticity) but also through transgenerational plasticity, providing lasting effects that are transmitted to the next generations (Donelson et al. 2012; Munday et al. 2013; Kuijper & Hoyle 2015; Auge et al. 2017, Tariel et al. 2020). These parental effects can affect offspring via various mechanisms, notably via maternal transmission of hormones to the eggs or growing embryos (Mousseau & Fox 1998). While the effects of environmental quality may simply carry-over to the next generation (e.g., females in stressful environments give birth to offspring in poorer condition), parental effects may also be a mechanism that adjusts offspring phenotype in response to environmental variation and predictability, and thereby match offspring's phenotype to future environmental conditions (Gluckman et al. 2005; Marshall & Uller 2007; Dey et al. 2016; Yin et al. 2019), for example by preparing their offspring to an expected stressful environment. When females experience stress during gestation or egg formation, elevations in glucocorticoids (GC) are expected to affect offspring phenotype in many ways, from the offspring's own GC levels, to their growth and survival (Sheriff et al. 2017). This is a well established idea, but how strong is the evidence for this? A meta-analysis on birds found no clear effect of corticosterone manipulation on offspring traits (38 studies on 9 bird species for corticosterone manipulation; Podmokła et al. 2018). Another meta-analysis including 14 vertebrate species found no clear effect of prenatal stress on offspring GC (Thayer et al. 2018). Finally, a meta-analysis on wild vertebrates (23 species) found no clear effect of GC-mediated maternal effects on offspring traits (MacLeod et al. 2021). As often when facing such inconclusive results, context dependence has been suggested as one potential reason for such inconsistencies, for exemple sex specific effects (Groothuis et al. 2019, 2020). However, sex specific measures on offspring are scarce (Podmokła et al. 2018). Moreover, the literature available is still limited to a few, mostly “model” species. With their study, Amin et al. (2024) show the way to improve our understanding on GC transmission from mother to offspring and its effects in several aspects. First they used innovative non-invasive methods (which could broaden the range of species available to study) by quantifying cortisol metabolites from faecal samples collected from pregnant females, as proxy for maternal GC level, and relating it to GC levels from hairs of their neonate offspring. Second they used a free ranging large mammal (taxa from which literature is missing): the fallow deer (Dama dama). Third, they provide sex specific measures of GC levels. And finally but importantly, they are exemplary in their transparency regarding 1) the exploratory nature of their study, 2) their statistical thinking and procedure, and 3) the study limitations (e.g., low sample size and high within individual variation of measurements). I hope this study will motivate more research (on the fallow deer, and on other species) to broaden and strengthen our understanding of sex specific effects of maternal stress and CG levels on offspring phenotype and fitness. References Amin, B., Fishman, R., Quinn, M., Matas, D., Palme, R., Koren, L., & Ciuti, S. (2024). Sex differences in the relationship between maternal and foetal glucocorticoids in a free-ranging large mammal. bioRxiv, ver. 4 peer-reviewed and recommended by Peer Community in Ecology. https://doi.org/10.1101/2023.05.04.538920 Auge, G.A., Leverett, L.D., Edwards, B.R. & Donohue, K. (2017). Adjusting phenotypes via within-and across-generational plasticity. New Phytologist, 216, 343–349. https://doi.org/10.1111/nph.14495 Dey, S., Proulx, S.R. & Teotonio, H. (2016). Adaptation to temporally fluctuating environments by the evolution of maternal effects. PLoS biology, 14, e1002388. https://doi.org/10.1371/journal.pbio.1002388 Donelson, J.M., Munday, P.L., McCormick, M.I. & Pitcher, C.R. (2012). Rapid transgenerational acclimation of a tropical reef fish to climate change. Nature Climate Change, 2, 30. https://doi.org/10.1038/nclimate1323 Gluckman, P.D., Hanson, M.A. & Spencer, H.G. (2005). Predictive adaptive responses and human evolution. Trends in ecology & evolution, 20, 527–533. https://doi.org/10.1016/j.tree.2005.08.001 Groothuis, Ton GG, Bin-Yan Hsu, Neeraj Kumar, and Barbara Tschirren. "Revisiting mechanisms and functions of prenatal hormone-mediated maternal effects using avian species as a model." Philosophical Transactions of the Royal Society B 374, no. 1770 (2019): 20180115. https://doi.org/10.1098/rstb.2018.0115 Groothuis, Ton GG, Neeraj Kumar, and Bin-Yan Hsu. "Explaining discrepancies in the study of maternal effects: the role of context and embryo." Current Opinion in Behavioral Sciences 36 (2020): 185-192. https://doi.org/10.1016/j.cobeha.2020.10.006 Kuijper, B. & Hoyle, R.B. (2015). When to rely on maternal effects and when on phenotypic plasticity? Evolution, 69, 950–968. https://doi.org/10.1111/evo.12635 MacLeod, Kirsty J., Geoffrey M. While, and Tobias Uller. "Viviparous mothers impose stronger glucocorticoid‐mediated maternal stress effects on their offspring than oviparous mothers." Ecology and Evolution 11, no. 23 (2021): 17238-17259. Marshall, D.J. & Uller, T. (2007). When is a maternal effect adaptive? Oikos, 116, 1957–1963. https://doi.org/10.1111/j.2007.0030-1299.16203.x Mousseau, T.A. & Fox, C.W. (1998). Maternal effects as adaptations. Oxford University Press. Munday, P.L., Warner, R.R., Monro, K., Pandolfi, J.M. & Marshall, D.J. (2013). Predicting evolutionary responses to climate change in the sea. Ecology Letters, 16, 1488–1500. https://doi.org/10.1111/ele.12185 Podmokła, Edyta, Szymon M. Drobniak, and Joanna Rutkowska. "Chicken or egg? Outcomes of experimental manipulations of maternally transmitted hormones depend on administration method–a meta‐analysis." Biological Reviews 93, no. 3 (2018): 1499-1517. https://doi.org/10.1111/brv.12406 Sheriff, M. J., Bell, A., Boonstra, R., Dantzer, B., Lavergne, S. G., McGhee, K. E., MacLeod, K. J., Winandy, L., Zimmer, C., & Love, O. P. (2017). Integrating ecological and evolutionary context in the study of maternal stress. Integrative and Comparative Biology, 57(3), 437–449. https://doi.org/10.1093/icb/icx105 Tariel, Juliette, Sandrine Plénet, and Émilien Luquet. "Transgenerational plasticity in the context of predator-prey interactions." Frontiers in Ecology and Evolution 8 (2020): 548660. https://doi.org/10.3389/fevo.2020.548660 Thayer, Zaneta M., Meredith A. Wilson, Andrew W. Kim, and Adrian V. Jaeggi. "Impact of prenatal stress on offspring glucocorticoid levels: A phylogenetic meta-analysis across 14 vertebrate species." Scientific Reports 8, no. 1 (2018): 4942. https://doi.org/10.1038/s41598-018-23169-w Yin, J., Zhou, M., Lin, Z., Li, Q.Q. & Zhang, Y.-Y. (2019). Transgenerational effects benefit offspring across diverse environments: a meta-analysis in plants and animals. Ecology letters, 22, 1976–1986. https://doi.org/10.1111/ele.13373 | Sex differences in the relationship between maternal and neonate cortisol in a free-ranging large mammal | Amin, B., Fishman, R., Quinn, M., Matas, D., Palme, R., Koren, L., Ciuti, S. | <p style="text-align: justify;">Maternal phenotypes can have long-term effects on offspring phenotypes. These maternal effects may begin during gestation, when maternal glucocorticoid (GC) levels may affect foetal GC levels, thereby having an orga... | Evolutionary ecology, Maternal effects, Ontogeny, Physiology, Zoology | Matthieu Paquet | 2023-06-05 09:06:56 | View | ||
19 Mar 2024
![]() How does dispersal shape the genetic patterns of animal populations in European cities? A simulation approachPaul Savary, Cécile Tannier, Jean-Christophe Foltête, Marc Bourgeois, Gilles Vuidel, Aurélie Khimoun, Hervé Moal, Stéphane Garnier https://doi.org/10.32942/X2JS41Gene flow in the city. Unravelling the mechanisms behind the variability in urbanization effects on genetic patterns.Recommended by Aurélie CoulonWorldwide, city expansion is happening at a fast rate and at the same time, urbanists are more and more required to make place for biodiversity. Choices have to be made regarding the area and spatial arrangement of suitable spaces for non-human living organisms, that will favor the long-term survival of their populations. To guide those choices, it is necessary to understand the mechanisms driving the effects of land management on biodiversity. Research results on the effects of urbanization on genetic diversity have been very diverse, with studies showing higher genetic diversity in rural than in urban populations (e.g. Delaney et al. 2010), the contrary (e.g. Miles et al. 2018) or no difference (e.g. Schoville et al. 2013). The same is true for studies investigating genetic differentiation. The reasons for these differences probably lie in the relative intensities of gene flow and genetic drift in each case study, which are hard to disentangle and quantify in empirical datasets. In their paper, Savary et al. (2024) used an elegant and powerful simulation approach to better understand the diversity of observed patterns and investigate the effects of dispersal limitation on genetic patterns (diversity and differentiation). Their simulations involved the landscapes of 325 real European cities, each under three different scenarios mimicking 3 virtual urban tolerant species with different abilities to move within cities while genetic drift intensity was held constant across scenarios. The cities were chosen so that the proportion of artificial areas was held constant (20%) but their location and shape varied. This design allowed the authors to investigate the effect of connectivity and spatial configuration of habitat on the genetic responses to spatial variations in dispersal in cities. The main results of this simulation study demonstrate that variations in dispersal spatial patterns, for a given level of genetic drift, trigger variations in genetic patterns. Genetic diversity was lower and genetic differentiation was larger when species had more difficulties to move through the more hostile components of the urban environment. The increase of the relative importance of drift over gene flow when dispersal was spatially more constrained was visible through the associated disappearance of the pattern of isolation by resistance. Forest patches (usually located at the periphery of the cities) usually exhibited larger genetic diversity and were less differentiated than urban green spaces. But interestingly, the presence of habitat patches at the interface between forest and urban green spaces lowered those differences through the promotion of gene flow. One other noticeable result, from a landscape genetic method point of view, is the fact that there might be a limit to the detection of barriers to genetic clusters through clustering analyses because of the increased relative effect of genetic drift. This result needs to be confirmed, though, as genetic structure has only been investigated with a recent approach based on spatial graphs. It would be interesting to also analyze those results with the usual Bayesian genetic clustering approaches. Overall, this study addresses an important scientific question about the mechanisms explaining the diversity of observed genetic patterns in cities. But it also provides timely cues for connectivity conservation and restoration applied to cities. Delaney, K. S., Riley, S. P., and Fisher, R. N. (2010). A rapid, strong, and convergent genetic response to urban habitat fragmentation in four divergent and widespread vertebrates. PLoS ONE, 5(9):e12767. | How does dispersal shape the genetic patterns of animal populations in European cities? A simulation approach | Paul Savary, Cécile Tannier, Jean-Christophe Foltête, Marc Bourgeois, Gilles Vuidel, Aurélie Khimoun, Hervé Moal, Stéphane Garnier | <p><em>Context and objectives</em></p> <p>Although urbanization is a major driver of biodiversity erosion, it does not affect all species equally. The neutral genetic structure of populations in a given species is affected by both genetic drift a... | ![]() | Biodiversity, Conservation biology, Dispersal & Migration, Eco-evolutionary dynamics, Human impact, Landscape ecology, Molecular ecology, Population ecology, Spatial ecology, Metacommunities & Metapopulations, Terrestrial ecology | Aurélie Coulon | 2023-07-25 19:09:16 | View | |
23 Jan 2024
![]() Use of linear features by red-legged partridges in an intensive agricultural landscape: implications for landscape management in farmlandCharlotte Perrot, Antoine Berceaux, Mathias Noel, Beatriz Arroyo, Leo Bacon https://doi.org/10.1101/2023.07.27.550774The importance of managing linear features in agricultural landscapes for farmland birdsRecommended by Ricardo Correia based on reviews by Matthew Grainger and 1 anonymous reviewerEuropean farmland bird populations continue declining at an alarming rate, and some species require urgent action to avoid their demise (Silva et al. 2024). While factors such as climate change and urbanization also play an important role in driving the decline of farmland bird populations, its main driver seems to be linked with agricultural intensification (Rigal et al. 2023). Besides increased pesticide and fertilizer use, agricultural intensification often results in the homogenization of agricultural landscapes through the removal of seminatural linear features such as hedgerows, field margins, and grassy strips that can be beneficial for biodiversity. These features may be particularly important during the breeding season, when breeding farmland birds can benefit from patches of denser vegetation to conceal nests and improve breeding success. It is both important and timely to understand how landscape management can help to address the ongoing decline of farmland birds by identifying specific actions that can boost breeding success. Perrot et al. 2023 contribute to this effort by exploring how red-legged partridges use linear features in an intensive agricultural landscape during the breeding season. Through a combination of targeted fieldwork and GPS tracking, the authors highlight patterns in home range size and habitat selection that provide insights for landscape management. Specifically, their results suggest that birds have smaller range sizes in the vicinity of traffic routes and seminatural features structured by both herbaceous and woody cover. Furthermore, they show that breeding birds tend to choose linear elements with herbaceous cover whereas non-breeders prefer linear elements with woody cover, underlining the importance of accounting for the needs of both breeding and non-breeding birds. In particular, the authors stress the importance of providing additional vegetation elements such as hedges, grassy strips or embankments in order to increase landscape heterogeneity. These landscape elements are usually found in the vicinity of linear infrastructures such as roads and tracks, but it is important they are available also in separate areas to avoid the risk of bird collision and the authors provide specific recommendations towards this end. Overall, this is an important study with clear recommendations on how to improve landscape management for these farmland birds. References Perrot, C., Séranne, L., Berceaux, A., Noel, M., Arroyo, B., & Bacon, L. (2023) "Use of linear features by red-legged partridges in an intensive agricultural landscape: implications for landscape management in farmland." bioRxiv, ver. 2 peer-reviewed and recommended by Peer Community in Ecology. | Use of linear features by red-legged partridges in an intensive agricultural landscape: implications for landscape management in farmland | Charlotte Perrot, Antoine Berceaux, Mathias Noel, Beatriz Arroyo, Leo Bacon | <p>Current agricultural practices and change are the major cause of biodiversity loss. An important change associated with the intensification of agriculture in the last 50 years is the spatial homogenization of the landscape with substantial loss... | ![]() | Agroecology, Behaviour & Ethology, Biodiversity, Conservation biology, Habitat selection | Ricardo Correia | 2023-08-01 10:27:33 | View | |
12 Jan 2024
![]() Methods for tagging an ectoparasite, the salmon louse Lepeophtheirus salmonisAlexius Folk, Adele Mennerat https://doi.org/10.1101/2023.08.31.555695Marking invertebrates using RFID tagsRecommended by Nicolas SchtickzelleGuiding and monitoring the efficiency of conservation efforts needs robust scientific background information, of which one key element is estimating wildlife abundance and its spatial and temporal variation. As raw counts are by nature incomplete counts of a population, correcting for detectability is required (Clobert, 1995; Turlure et al., 2018). This can be done with Capture-Mark-Recapture protocols (Iijima, 2020). Techniques for marking individuals are diverse, e.g. writing on butterfly wings, banding birds, or using natural specific patterns in the individual’s body such as leopard fur or whale tail. Advancement in technology opens new opportunities for developing marking techniques, including strategies to limit mark identification errors (Burchill & Pavlic, 2019), and for using active marks that can transmit data remotely or be read automatically. The details of such methodological developments frequently remain unpublished, the method being briefly described in studies that use it. For a few years, there has been however a renewed interest in proper publishing of methods for ecology and evolution. This study by Folk & Mennerat (2023) fits in this context, offering a nice example of detailed description and testing of a method to mark salmon ectoparasites using RFID tags. Such tags are extremely small, yet easy to use, even with automatic recording procedure. The study provides a very good basis protocol that should help researchers working for small species, in particular invertebrates. The study is complemented by a video illustrating the placement of the tag so the reader who would like to replicate the procedure can get a very precise idea of it. References Burchill, A. T., & Pavlic, T. P. (2019). Dude, where’s my mark? Creating robust animal identification schemes informed by communication theory. Animal Behaviour, 154, 203–208. https://doi.org/10.1016/j.anbehav.2019.05.013 Clobert, J. (1995). Capture-recapture and evolutionary ecology: A difficult wedding ? Journal of Applied Statistics, 22(5–6), 989–1008. Folk, A., & Mennerat, A. (2023). Methods for tagging an ectoparasite, the salmon louse Lepeophtheirus salmonis (p. 2023.08.31.555695). bioRxiv, ver. 2 peer-reviewed and recommended by Peer Community in Ecology. https://doi.org/10.1101/2023.08.31.555695 Iijima, H. (2020). A Review of Wildlife Abundance Estimation Models: Comparison of Models for Correct Application. Mammal Study, 45(3), 177–188. https://doi.org/10.3106/ms2019-0082 Turlure, C., Pe’er, G., Baguette, M., & Schtickzelle, N. (2018). A simplified mark–release–recapture protocol to improve the cost effectiveness of repeated population size quantification. Methods in Ecology and Evolution, 9(3), 645–656. https://doi.org/10.1111/2041-210X.12900 | Methods for tagging an ectoparasite, the salmon louse *Lepeophtheirus salmonis* | Alexius Folk, Adele Mennerat | <p style="text-align: justify;">Monitoring individuals within populations is a cornerstone in evolutionary ecology, yet individual tracking of invertebrates and particularly parasitic organisms remains rare. To address this gap, we describe here a... | ![]() | Dispersal & Migration, Evolutionary ecology, Host-parasite interactions, Marine ecology, Parasitology, Terrestrial ecology, Zoology | Nicolas Schtickzelle | 2023-09-04 15:25:08 | View | |
15 Feb 2024
![]() Sources of confusion in global biodiversity trendsMaelys Boennec, Vasilis Dakos, Vincent Devictor https://doi.org/10.32942/X29W3HUnraveling the Complexity of Global Biodiversity Dynamics: Insights and ImperativesRecommended by Paulo BorgesBiodiversity loss is occurring at an alarming rate across terrestrial and marine ecosystems, driven by various processes that degrade habitats and threaten species with extinction. Despite the urgency of this issue, empirical studies present a mixed picture, with some indicating declining trends while others show more complex patterns. In a recent effort to better understand global biodiversity dynamics, Boennec et al. (2024) conducted a comprehensive literature review examining temporal trends in biodiversity. Their analysis reveals that reviews and meta-analyses, coupled with the use of global indicators, tend to report declining trends more frequently. Additionally, the study underscores a critical gap in research: the scarcity of investigations into the combined impact of multiple pressures on biodiversity at a global scale. This lack of understanding complicates efforts to identify the root causes of biodiversity changes and develop effective conservation strategies. This study serves as a crucial reminder of the pressing need for long-term biodiversity monitoring and large-scale conservation studies. By filling these gaps in knowledge, researchers can provide policymakers and conservation practitioners with the insights necessary to mitigate biodiversity loss and safeguard ecosystems for future generations. References Boennec, M., Dakos, V. & Devictor, V. (2023). Sources of confusion in global biodiversity trend. bioRxiv, ver. 4 peer-reviewed and recommended by Peer Community in Ecology. https://doi.org/10.32942/X29W3H
| Sources of confusion in global biodiversity trends | Maelys Boennec, Vasilis Dakos, Vincent Devictor | <p>Populations and ecological communities are changing worldwide, and empirical studies exhibit a mixture of either declining or mixed trends. Confusion in global biodiversity trends thus remains while assessing such changes is of major social, po... | ![]() | Biodiversity, Conservation biology, Meta-analyses | Paulo Borges | 2023-09-20 11:10:25 | View | |
13 May 2024
![]() Getting More by Asking for Less: Linking Species Interactions to Species Co-Distributions in MetacommunitiesMatthieu Barbier, Guy Bunin, Mathew A. Leibold https://doi.org/10.1101/2023.06.04.543606Beyond pairwise species interactions: coarser inference of their joined effects is more relevantRecommended by François MunozBarbier et al. (2024) investigated the dynamics of species abundances depending on their ecological niche (abiotic component) and on (numerous) competitive interactions. In line with previous evidence and expectations (Barbier et al. 2018), the authors show that it is possible to robustly infer the mean and variance of interaction coefficients from species co-distributions, while it is not possible to infer the individual coefficient values. The authors devised a simulation framework representing multispecies dynamics in an heterogeneous environmental context (2D grid landscape). They used a Lotka-Volterra framework involving pairwise interaction coefficients and species-specific carrying capacities. These capacities depend on how well the species niche matches the local environmental conditions, through a Gaussian function of the distance of the species niche centers to the local environmental values. They considered two contrasted scenarios denoted as « Environmental tracking » and « Dispersal limited ». In the latter case, species are initially seeded over the environmental grid and cannot disperse to other cells, while in the former case they can disperse and possibly be more performant in other cells. The direct effects of species on one another are encoded in an interaction matrix A, and the authors further considered net interactions depending on the inverse of the matrix of direct interactions (Zelnik et al., 2024). The net effects are context-dependent, i.e., it involves the environment-dependent biotic capacities, even through the interaction terms can be defined between species as independent from local environment. The results presented here underline that the outcome of many individual competitive interactions can only be understood in terms of macroscopic properties. In essence, the results here echoe the mean field theories that investigate the dynamics of average ecological properties instead of the microscopic components (e.g., McKane et al. 2000). In a philosophical perspective, community ecology has long struggled with analyzing and inferring local determinants of species coexistence from species co-occurrence patterns, so that it was claimed that no universal laws can be derived in the discipline (Lawton 1999). Using different and complementary methods and perspectives, recent research has also shown that species assembly parameter values cannot be unambiguously inferred from species co-occurrences only, even in simple designs where an equilibrium can be reached (Poggiato et al. 2021). Although the roles of high-order competitive interactions and intransivity can lead to species coexistence, the simple view of a single loop of competitive interactions is easily challenged when further interactions and complexity is added (Gallien et al. 2024). But should we put so much emphasis on inferring individual interaction coefficients? In a quest to understand the emerging properties of elementary processes, ecological theory could go forward with a more macroscopic analysis and understanding of species coexistence in many communities. The authors referred several times to an interesting paper from Schaffer (1981), entitled « Ecological abstraction: the consequences of reduced dimensionality in ecological models ». It proposes that estimating individual species competition coefficients is not possible, but that competition can be assessed at the coarser level of organisation, i.e., between ecological guilds. This idea implies that the dimensionality of the competition equations should be greatly reduced to become tractable in practice. Taking together this claim with the results of the present Barbier et al. (2024) paper, it becomes clearer that the nature of competitive interactions can be addressed through « abstracted » quantities, as those of guilds or the moments of the individual competition coefficients (here the average and the standard deviation). Therefore the scope of Barbier et al. (2024) framework goes beyond statistical issues in parameter inference, but question the way we must think and represent the numerous competitive interactions in a simplified and robust way. References Barbier, Matthieu, Jean-François Arnoldi, Guy Bunin, et Michel Loreau. 2018. « Generic assembly patterns in complex ecological communities ». Proceedings of the National Academy of Sciences 115 (9): 2156‑61. https://doi.org/10.1073/pnas.1710352115 | Getting More by Asking for Less: Linking Species Interactions to Species Co-Distributions in Metacommunities | Matthieu Barbier, Guy Bunin, Mathew A. Leibold | <p>AbstractOne of the more difficult challenges in community ecology is inferring species interactions on the basis of patterns in the spatial distribution of organisms. At its core, the problem is that distributional patterns reflect the ‘realize... | ![]() | Biogeography, Community ecology, Competition, Spatial ecology, Metacommunities & Metapopulations, Species distributions, Statistical ecology, Theoretical ecology | François Munoz | 2023-10-21 14:14:16 | View | |
20 Jun 2024
![]() Spider mites collectively avoid plants with cadmium irrespective of their frequency or the presence of competitorsDiogo Prino Godinho*, Ines Fragata*, Maud Charlery de la Masseliere, Sara Magalhaes https://doi.org/10.1101/2023.08.17.553707We are better together: Spider mites running away from Cadmium contaminated plants make better decisions collectively than individuallyRecommended by Ruben HelenoHyperaccumulator plants can concentrate heavy metals present on the soil in their tissues, avoiding their toxic effects and potentially discouraging herbivores (Martens & Boyd, 1994). But not all herbivores are necessarily discouraged, and access to locally abundant resources with low interspecific competition from other herbivores, can affect feeding choices. Godinho et al. performed a series of controlled laboratorial trials to evaluate if herbivores (spider mites) avoid tomato plants with high concentrations of Cadmium under alternative scenarios, namely: the presence/absence of conspecifics, the presence/absence of a competitor species (a congeneric mite), and the relative abundance of contaminated plants. They found that when looking for plants to lay their eggs, individual spider-mites (females) do not seem to discriminate between plants with or without cadmium, despite a significantly lower performance on the former. However, they consistently chose plants without Cadmium in set-ups where 200 mites are faced with this decision together. This preference was consistent and independent from the relative abundance of cadmium-free plants, but only when mites do this decision collectively. In addition, this preference was stronger than that for plants where interspecific competition was lower, with mites preferring to face high competition from congeneric herbivores than laying their eggs on Cadmium contaminated plants. Taken together these experiments suggest that aggregation is a key mechanism by which spider mites can avoid metal contaminated plants. As good research often does, these experiments open several important questions that will need to be addressed in the future. In particular, it will be important to clarify what are the sensorial and behavioural mechanisms that allow this decision/outcome when spider mites make this choice collectively but lead to a different outcome (no choice) when they face this decision alone. Additionally, it will be interesting to explore the potentially adaptive (or non-adaptive) consequences of this behaviour in terms of individual and inclusive fitness. One thing seems certain: both the abiotic and the biotic context can affect spider mite choices, and both need to be considered to advance our understanding about the trade-offs between plant defence mechanisms and associated herbivore decisions and fitness. References Martens, S. N., & Boyd, R. S. (1994). The ecological significance of nickel hyperaccumulation: a plant chemical defense. Oecologia, 98(3–4), 379–384. https://doi.org/10.1007/BF00324227 Godinho, D. P., I. Fragata, M. C. de la Masseliere, S. Magalhaes 2024 Spider mites collectively avoid plants with cadmium irrespective of their frequency or the presence of competitors. bioRxiv, ver. 4, peer-reviewed and recommended by PCI Ecology 2023.08.17.553707. https://doi.org/10.1101/2023.08.17.553707
| Spider mites collectively avoid plants with cadmium irrespective of their frequency or the presence of competitors | Diogo Prino Godinho*, Ines Fragata*, Maud Charlery de la Masseliere, Sara Magalhaes | <p>1. Plants can accumulate heavy metals from polluted soils on their shoots and use this to defend themselves against herbivory. One possible strategy for herbivores to cope with the reduction in performance imposed by heavy metal accumulation in... | ![]() | Behaviour & Ethology, Competition, Habitat selection, Herbivory | Ruben Heleno | 2023-11-09 11:52:58 | View | |
18 Apr 2024
![]() The large and central Caligo martia eyespot may reduce fatal attacks by birds: a case study supports the deflection hypothesis in natureCristiano Agra Iserhard, Shimene Torve Malta, Carla Maria Penz, Brenda Barbon Fraga, Camila Abel da Costa, Taiane Schwantz, Kauane Maiara Bordin https://doi.org/10.5281/zenodo.10980357Intimidation or deflection: field experiments in a tropical forest to simultaneously test two competing hypotheses about how butterfly eyespots confer protection against predatorsRecommended by Doyle Mc KeyEyespots—round or oval spots, usually accompanied by one or more concentric rings, that together imitate vertebrate eyes—are found in insects of at least three orders and in some tropical fishes (Stevens 2005). They are particularly frequent in Lepidoptera, where they occur on wings of adults in many species (Monteiro et al. 2006), and in caterpillars of many others (Janzen et al. 2010). The resemblance of eyespots to vertebrate eyes often extends to details, such as fake « pupils » (round or slit-like) and « eye sparkle » (Blut et al. 2012). Larvae of one hawkmoth species even have fake eyes that appear to blink (Hossie et al. 2013). Eyespots have interested evolutionary biologists for well over a century. While they appear to play a role in mate choice in some adult Lepidoptera, their adaptive significance in adult Lepidoptera, as in caterpillars, is mainly as an anti-predator defense (Monteiro 2015). However, there are two competing hypotheses about the mechanism by which eyespots confer defense against predators. The « intimidation » hypothesis postulates that eyespots intimidate potential predators, startling them and reducing the probability of attack. The « deflection » hypothesis holds that eyespots deflect attacks to parts of the body where attack has relatively little effect on the animal’s functioning and survival. In caterpillars, there is little scope for the deflection hypothesis, because attack on any part of a caterpillar’s body is likely to be lethal. Much observational and some experimental evidence supports the intimidation hypothesis in caterpillars (Hossie & Sherratt 2012). In adult Lepidoptera, however, both mechanisms are plausible, and both have found support (Stevens 2005). The most spectacular examples of intimidation are in butterflies in which eyespots located centrally in hindwings and hidden in the natural resting position are suddenly exposed, startling the potential predator (e.g., Vallin et al. 2005). The most spectacular examples of deflection are seen in butterflies in which eyespots near the hindwing margin combined with other traits give the appearance of a false head (e.g., Chotard et al. 2022; Kodandaramaiah 2011). Most studies have attempted to test for only one or the other of these mechanisms—usually the one that seems a priori more likely for the butterfly species being studied. But for many species, particularly those that have neither spectacular startle displays nor spectacular false heads, evidence for or against the two hypotheses is contradictory. Iserhard et al. (2024) attempted to simultaneously test both hypotheses, using the neotropical nymphalid butterfly Caligo martia. This species has a large ventral hindwing eyespot, exposed in the insect’s natural resting position, while the rest of the ventral hindwing surface is cryptically coloured. In a previous study of this species, De Bona et al. (2015) presented models with intact and disfigured eyespots on a computer monitor to a European bird species, the great tit (Parus major). The results favoured the intimidation hypothesis. Iserhard et al. (2024) devised experiments presenting more natural conditions, using fairly realistic dummy butterflies, with eyespots manipulated or unmanipulated, exposed to a diverse assemblage of insectivorous birds in nature, in a tropical forest. Using color-printed paper facsimiles of wings, with eyespots present, UV-enhanced, or absent, they compared the frequency of beakmarks on modeling clay applied to wing margins (frequent attacks would support the deflection hypothesis) and (in one of two experiments) on dummies with a modeling-clay body (eyespots should lead to reduced frequency of attack, to wings and body, if birds are intimidated). Their experiments also included dummies without eyespots whose wings were either cryptically coloured (as in unmanipulated butterflies) or not. Their results, although complex, indicate support for the deflection hypothesis: dummies with eyespots were mostly attacked on these less vital parts. Dummies lacking eyespots were less frequently attacked, especially when they were camouflaged. Camouflaged dummies without eyespots were in fact the least frequently attacked of all the models. However, when dummies lacking eyespots were attacked, attacks were usually directed to vital body parts. These results show some of the complexity of estimating costs and benefits of protective conspicuous signals vs. camouflage (Stevens et al. 2008). Two complementary experiments were conducted. The first used facsimiles with « wings » in a natural resting position (folded, ventral surfaces exposed), but without a modeling-clay « body ». In the second experiment, facsimiles had a modeling-clay « body », placed between the two unfolded wings to make it as accessible to birds as the wings. However, these dummies displayed the ventral surfaces of unfolded wings, an unnatural resting position. The study was thus not able to compare bird attacks to the body vs. wings in a natural resting position. One can understand the reason for this methodological choice, but it is a limitation of the study. The naturalness of the conditions under which these field experiments were conducted is a strong argument for the biological significance of their results. However, the uncontrolled conditions naturally result in many questions being left open. The butterfly dummies were exposed to at least nine insectivorous bird species. Do bird species differ in their behavioral response to eyespots? Do responses depend on the distance at which a bird first detects the butterfly? Do eyespots and camouflage markings present on the same animal both function, but at different distances (Tullberg et al. 2005)? Do bird responses vary depending on the particular light environment in the places and at the times when they encounter the butterfly (Kodandaramaiah 2011)? Answering these questions under natural, uncontrolled conditions will be challenging, requiring onerous methods, (e.g., video recording in multiple locations over time). The study indicates the interest of pursuing these questions. References Blut, C., Wilbrandt, J., Fels, D., Girgel, E.I., & Lunau, K. (2012). The ‘sparkle’ in fake eyes–the protective effect of mimic eyespots in Lepidoptera. Entomologia Experimentalis et Applicata, 143, 231-244. https://doi.org/10.1111/j.1570-7458.2012.01260.x Chotard, A., Ledamoisel, J., Decamps, T., Herrel, A., Chaine, A.S., Llaurens, V., & Debat, V. (2022). Evidence of attack deflection suggests adaptive evolution of wing tails in butterflies. Proceedings of the Royal Society B, 289, 20220562. https://doi.org/10.1098/rspb.2022.0562 De Bona, S., Valkonen, J.K., López-Sepulcre, A., & Mappes, J. (2015). Predator mimicry, not conspicuousness, explains the efficacy of butterfly eyespots. Proceedings of the Royal Society B, 282, 1806. https://doi.org/10.1098/RSPB.2015.0202 Hossie, T.J., & Sherratt, T.N. (2012). Eyespots interact with body colour to protect caterpillar-like prey from avian predators. Animal Behaviour, 84, 167-173. https://doi.org/10.1016/j.anbehav.2012.04.027 Hossie, T.J., Sherratt, T.N., Janzen, D.H., & Hallwachs, W. (2013). An eyespot that “blinks”: an open and shut case of eye mimicry in Eumorpha caterpillars (Lepidoptera: Sphingidae). Journal of Natural History, 47, 2915-2926. https://doi.org/10.1080/00222933.2013.791935 Iserhard, C.A., Malta, S.T., Penz, C.M., Brenda Barbon Fraga; Camila Abel da Costa; Taiane Schwantz; & Kauane Maiara Bordin (2024). The large and central Caligo martia eyespot may reduce fatal attacks by birds : a case study supports the deflection hypothesis in nature. Zenodo, ver. 1 peer-reviewed and recommended by Peer Community in Ecology. https://doi.org/10.5281/zenodo.10980357 Janzen, D.H., Hallwachs, W., & Burns, J.M. (2010). A tropical horde of counterfeit predator eyes. Proceedings of the National Academy of Sciences, USA, 107, 11659-11665. https://doi.org/10.1073/pnas.0912122107 Kodandaramaiah, U. (2011). The evolutionary significance of butterfly eyespots. Behavioral Ecology, 22, 1264-1271. https://doi.org/10.1093/beheco/arr123 Monteiro, A. (2015). Origin, development, and evolution of butterfly eyespots. Annual Review of Entomology, 60, 253-271. https://doi.org/10.1146/annurev-ento-010814-020942 Monteiro, A., Glaser, G., Stockslager, S., Glansdorp, N., & Ramos, D. (2006). Comparative insights into questions of lepidopteran wing pattern homology. BMC Developmental Biology, 6, 1-13. https://doi.org/10.1186/1471-213X-6-52 Stevens, M. (2005). The role of eyespots as anti-predator mechanisms, principally demonstrated in the Lepidoptera. Biological Reviews, 80, 573–588. https://doi.org/10.1017/S1464793105006810 Stevens, M., Stubbins, C.L., & Hardman C.J. (2008). The anti-predator function of ‘eyespots’ on camouflaged and conspicuous prey. Behavioral Ecology and Sociobiology, 62, 1787-1793. https://doi.org/10.1007/s00265-008-0607-3 Tullberg, B.S., Merilaita, S., & Wiklund, C. (2005). Aposematism and crypsis combined as a result of distance dependence: functional versatility of the colour pattern in the swallowtail butterfly larva. Proceedings of the Royal Society B, 272, 1315-1321. https://doi.org/10.1098/rspb.2005.3079 Vallin, A., Jakobsson, S., Lind, J., & Wiklund, C. (2005). Prey survival by predator intimidation: an experimental study of peacock butterfly defence against blue tits. Proceedings of the Royal Society B, 272, 1203-1207. https://doi.org/10.1098/rspb.2004.3034 | The large and central *Caligo martia* eyespot may reduce fatal attacks by birds: a case study supports the deflection hypothesis in nature | Cristiano Agra Iserhard, Shimene Torve Malta, Carla Maria Penz, Brenda Barbon Fraga, Camila Abel da Costa, Taiane Schwantz, Kauane Maiara Bordin | <p>Many animals have colorations that resemble eyes, but the functions of such eyespots are debated. Caligo martia (Godart, 1824) butterflies have large ventral hind wing eyespots, and we aimed to test whether these eyespots act to deflect or to t... | ![]() | Biodiversity, Community ecology, Conservation biology, Life history, Tropical ecology | Doyle Mc Key | 2023-11-21 15:00:20 | View | |
28 Mar 2024
![]() Changes in length-at-first return of a sea trout (Salmo trutta) population in northern FranceQuentin Josset, Laurent Beaulaton, Atso Romakkaniemi, Marie Nevoux https://doi.org/10.1101/2023.11.21.568009Why are trout getting smaller?Recommended by Aleksandra Walczyńska based on reviews by Jan Kozlowski and 1 anonymous reviewerDecline in body size over time have been widely observed in fish (but see Solokas et al. 2023), and the ecological consequences of this pattern can be severe (e.g., Audzijonyte et al. 2013, Oke et al. 2020). Therefore, studying the interrelationships between life history traits to understand the causal mechanisms of this pattern is timely and valuable. This phenomenon was the subject of a study by Josset et al. (2024), in which the authors analysed data from 39 years of trout trapping in the Bresle River in France. The authors focused mainly on the length of trout on their first return from the sea. The most important results of the study were the decrease in fish length-at-first return and the change in the age structure of first-returning trout towards younger (and earlier) returning fish. It seems then that the smaller size of trout is caused by a shorter time spent in the sea rather than a change in a growth pattern, as length-at-age remained relatively constant, at least for those returning earlier. Fish returning after two years spent in the sea had a relatively smaller length-at-age. The authors suggest this may be due to local changes in conditions during fish's stay in the sea, although there is limited environmental data to confirm the causal effect. Another question is why there are fewer of these older fish. The authors point to possible increased mortality from disease and/or overfishing. These results may suggest that the situation may be getting worse, as another study finding was that “the more growth seasons an individual spent at sea, the greater was its length-at-first return.” The consequences may be the loss of the oldest and largest individuals, whose disproportionately high reproductive contribution to the population is only now understood (Barneche et al. 2018, Marshall and White 2019). Audzijonyte, A. et al. 2013. Ecological consequences of body size decline in harvested fish species: positive feedback loops in trophic interactions amplify human impact. Biol Lett 9, 20121103. https://doi.org/10.1098/rsbl.2012.1103 Oke, K. B. et al. 2020. Recent declines in salmon body size impact ecosystems and fisheries. Nature Communications, 11, 4155. https://doi.org/10.1038/s41467-020-17726-z Solokas, M. A. et al. 2023. Shrinking body size and climate warming: many freshwater salmonids do not follow the rule. Global Change Biology, 29, 2478-2492. https://doi.org/10.1111/gcb.16626 | Changes in length-at-first return of a sea trout (*Salmo trutta*) population in northern France | Quentin Josset, Laurent Beaulaton, Atso Romakkaniemi, Marie Nevoux | <p style="text-align: justify;">The resilience of sea trout populations is increasingly concerning, with evidence of major demographic changes in some populations. Based on trapping data and related scale collection, we analysed long-term changes ... | ![]() | Biodiversity, Evolutionary ecology, Freshwater ecology, Life history, Marine ecology | Aleksandra Walczyńska | 2023-11-23 14:36:39 | View |
MANAGING BOARD
Julia Astegiano
Tim Coulson
Anna Eklof
Dominique Gravel
François Massol
Ben Phillips
Cyrille Violle