Field assessment of precocious maturation in salmon parr using ultrasound imaging
Marie Nevoux, Frédéric Marchand, Guillaume Forget, Dominique Huteau, Julien Tremblay, Jean-Pierre Destouches
https://doi.org/10.1101/425561
OB-GYN for salmon parrs
Recommended by Jean-Olivier Irisson based on reviews by Hervé CAPRA and 1 anonymous reviewer
Population dynamics and stock assessment models are only as good as the data used to parameterise them. For Atlantic salmon (Salmo salar) populations, a critical parameter may be frequency of precocious maturation. Indeed, the young males (parrs) that mature early, before leaving the river to reach the ocean, can contribute to reproduction but have much lower survival rates afterwards. The authors cite evidence of the potentially major consequences of this alternate reproductive strategy. So, to be parameterised correctly, it needs to be assessed correctly. Cue the ultrasound machine.
Through a thorough analysis of data collected on 850 individuals [1], over three years, the authors clearly show that the non-invasive examination of the internal cavity of young fishes to look for gonads, using a portable ultrasound machine, provides reliable and replicable evidence of precocious maturation. They turned into OB-GYN for salmons (albeit for male salmons!) and it worked. While using ultrasounds to detect fish gonads is not a new idea (early attempts for salmonids date back to the 80s [2]), the value here is in the comparison with the classic visual inspection technique (which turns out to be less reliable) and the fact that ultrasounds can now easily be carried out in the field.
Beyond the potentially important consequences of this new technique for the correct assessment of salmon population dynamics, the authors also make the case for the acquisition of more reliable individual-level data in ecological studies, which I applaud.
References.
[1] Nevoux M, Marchand F, Forget G, Huteau D, Tremblay J, and Destouches J-P. (2019). Field assessment of precocious maturation in salmon parr using ultrasound imaging. bioRxiv 425561, ver. 3 peer-reviewed and recommended by PCI Ecology. doi: 10.1101/425561
[2] Reimers E, Landmark P, Sorsdal T, Bohmer E, Solum T. (1987). Determination of salmonids’ sex, maturation and size: an ultrasound and photocell approach. Aquaculture Magazine.13:41-44.
| Field assessment of precocious maturation in salmon parr using ultrasound imaging | Marie Nevoux, Frédéric Marchand, Guillaume Forget, Dominique Huteau, Julien Tremblay, Jean-Pierre Destouches | <p>Salmonids are characterized by a large diversity of life histories, but their study is often limited by the imperfect observation of the true state of an individual in the wild. Challenged by the need to reduce uncertainty of empirical data, re... | 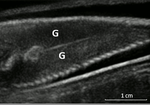 | Conservation biology, Demography, Experimental ecology, Freshwater ecology, Life history, Phenotypic plasticity, Population ecology | Jean-Olivier Irisson | | 2018-09-25 17:24:59 | View |
Probing behaviors correlated with behavioral flexibility
Recommended by Jeremy Van Cleve based on reviews by 2 anonymous reviewers
Behavioral plasticity, which is a subset of phenotypic plasticity, is an important component of foraging, defense against predators, mating, and many other behaviors. More specifically, behavioral flexibility, in this study, captures how quickly individuals adapt to new circumstances. In cases where individuals disperse to new environments, which often occurs in range expansions, behavioral flexibility is likely crucial to the chance that individuals can establish in these environments. Thus, it is important to understand how best to measure behavioral flexibility and how measures of such flexibility might vary across individuals and behavioral contexts and with other measures of learning and problem solving.
In this preregistration, Logan and colleagues propose to use a long-term study of the great-tailed grackle to measure how much they can manipulate behavioral flexibility in a reversal learning task, how much behavioral flexibility in one task predicts flexibility in another task and in problem solving a new task, and how robust these patterns are within individuals and across tasks. Logan and colleagues lay out their hypotheses and predictions for each experiment in a clear and concise manner. They also are very clear about the details of their study system, such as how they determined the number of trials they use in their learning reversal experiments, and how those details have influenced their experimental design. Further, given that the preregistration uses RMarkdown and is stored on GitHub (as are other studies in the larger project), their statistical code and its history of modification are easily available. This is a crucial component of making research more reproducible, which is a recent emphasis in behavioral sciences more broadly.
Reviewers of this preregistration found the study of substantial merit. The authors have responded to the reviewers' comments and their revisions have made the preregistration much clearer and cogent. I am happy to recommend this preregistration.
| Is behavioral flexibility linked with exploration, but not boldness, persistence, or motor diversity? | Kelsey McCune, Carolyn Rowney, Luisa Bergeron, Corina Logan | This is a PREREGISTRATION. The DOI was issued by OSF and refers to the whole GitHub repository, which contains multiple files. The specific file we are submitting is g_exploration.Rmd, which is easily accessible at GitHub at https://github.com/cor... | 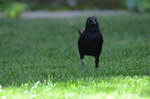 | Behaviour & Ethology, Preregistrations, Zoology | Jeremy Van Cleve | | 2018-09-27 03:35:12 | View |
The inherent multidimensionality of temporal variability: How common and rare species shape stability patterns
Jean-François Arnoldi, Michel Loreau, Bart Haegeman
https://doi.org/10.1101/431296
Diversity-Stability and the Structure of Perturbations
Recommended by Kevin Cazelles and Kevin Shear McCann based on reviews by Frederic Barraquand and 1 anonymous reviewer
In his 1972 paper “Will a Large Complex System Be Stable?” [1], May challenges the idea that large communities are more stable than small ones. This was the beginning of a fundamental debate that still structures an entire research area in ecology: the diversity-stability debate [2]. The most salient strength of May’s work was to use a mathematical argument to refute an idea based on the observations that simple communities are less stable than large ones. Using the formalism of dynamical systems and a major results on the distribution of the eigen values for random matrices, May demonstrated that the addition of random interactions destabilizes ecological communities and thus, rich communities with a higher number of interactions should be less stable. But May also noted that his mathematical argument holds true only if ecological interactions are randomly distributed and thus concluded that this must not be true! This is how the contradiction between mathematics and empirical observations led to new developments in the study of ecological networks.
Since 1972, the theoretical corpus of ecology has advanced, building on the formalism of dynamical systems, ecologists have revealed that ecological interactions are indeed not randomly distributed [3,4], but general rules are still missing and we are far from understanding what determine the exact network topology of a given community. One promising avenue is to understand the relationship between different facets of the concept of stability [5,6]. Indeed, the classical approach to determine whether a system is stable is qualitative: if a system returns to its equilibrium when it is slightly moved away from it, then the system is considered stable. But there are several other aspects that are worth scrutinizing. For instance, when a system returns to its equilibrium, one can characterize the corresponding transient dynamics [7,8], that is asking fundamental questions such as: what is the trajectory of return? How long does it take to return to the equilibrium? Another fundamental question is whether the system remains qualitatively stable when the distributions of interactions strengths change? From a biological standpoint, all of these questions matter as all these aspects of stability may partially explain the actual structure of ecological networks, and hence, frameworks that integrate several facets of stability are much needed.
The study by Arnoldi et al. [9] is a significant step towards such a framework. The strength of their formalism is threefold. First, instead of considering separately the system and its perturbations, they considering the fluctuations of a perturbed ecological systems and thus, perturbations are parts of the ecological system. Second, they use of a broad definition of perturbation that encompasses the types of perturbations (whether the individual respond synchronously or not), their intensity and their direction (how the perturbations are correlated across species). Third, they quantify the instability of the system using variability which integrates the consequences of perturbations over the whole set of species of a community: such a measure is comparable across communities and accounts for the trivial effect of the perturbations on the system dynamics.
Using this framework, the authors show that interactions within a stable community leads to a general relationship between variability and the abundance of individually perturbed species: if individuals of species respond in synchrony to a perturbation, then the more abundant the species perturbed the higher the variability of the system, but the relationship is reverse when individual respond asynchronously. A direct implications of these results for the classical debate is that the diversity-stability relationship is negative for the former type of perturbations (as in May’s seminal paper) but positive for the latter type. Hence, the rigorous work of Arnoldi and colleagues sheds a new light upon the classical debate: the nature of the perturbation regime prevailing within a community affects the slope of the diversity-stability relationships and given the vast diversity of ecological communities, this may very well be one of the reasons why the debate still endures.
From a historical perspective, it is interesting that ecologists have gone from looking at random webs to structured webs and now, in a sense, Arnoldi et al. are unpacking the role of differentially structured perturbations. The work they achieved will doubtlessly be followed by further theoretical investigations. One natural research avenue is to revisit the role of the topology of ecological networks with this framework: how the distribution of interactions and their strength affect the general relationship they unravel? Finally, this study demonstrate that the impact of the abundance of a species on the variability of the system depends on the nature of the perturbation regime and so the distribution of species abundances within a community should be determined by the prevailing perturbation regime which is a prediction that remains to be tested.
References
[1] May, Robert M (1972). Will a Large Complex System Be Stable? Nature 238, 413–414. doi: 10.1038/238413a0
[2] McCann, Kevin Shear (2000). The Diversity–Stability Debate. Nature 405, 228–233. doi: 10.1038/35012234
[3] Rooney, Neil, Kevin McCann, Gabriel Gellner, and John C. Moore (2006). Structural Asymmetry and the Stability of Diverse Food Webs. Nature 442, 265–269. doi: 10.1038/nature04887
[4] Jacquet, Claire, Charlotte Moritz, Lyne Morissette, Pierre Legagneux, François Massol, Philippe Archambault, and Dominique Gravel (2016). No Complexity–Stability Relationship in Empirical Ecosystems. Nature Communications 7, 12573. doi: 10.1038/ncomms12573
[5] Donohue, Ian, Helmut Hillebrand, José M. Montoya, Owen L. Petchey, Stuart L. Pimm, Mike S. Fowler, Kevin Healy, et al. (2016). Navigating the Complexity of Ecological Stability. Ecology Letters 19, 1172–1185. doi: 10.1111/ele.12648
[6] Arnoldi, Jean-François, and Bart Haegeman (2016). Unifying Dynamical and Structural Stability of Equilibria. Proceedings of the Royal Society A: Mathematical, Physical and Engineering Science 472, 20150874. doi: 10.1098/rspa.2015.0874
[7] Caswell, Hal, and Michael G. Neubert (2005). Reactivity and Transient Dynamics of Discrete-Time Ecological Systems. Journal of Difference Equations and Applications 11, 295–310. doi: 10.1080/10236190412331335382
[8] Arnoldi, J-F., M. Loreau, and B. Haegeman (2016). Resilience, Reactivity and Variability: A Mathematical Comparison of Ecological Stability Measures. Journal of Theoretical Biology 389, 47–59. doi: 10.1016/j.jtbi.2015.10.012
[9] Arnoldi, Jean-Francois, Michel Loreau, and Bart Haegeman. (2019). The Inherent Multidimensionality of Temporal Variability: How Common and Rare Species Shape Stability Patterns.” BioRxiv, 431296, ver. 3 peer-reviewed and recommended by PCI Ecology. doi: 10.1101/431296
| The inherent multidimensionality of temporal variability: How common and rare species shape stability patterns | Jean-François Arnoldi, Michel Loreau, Bart Haegeman | <p>Empirical knowledge of ecosystem stability and diversity-stability relationships is mostly based on the analysis of temporal variability of population and ecosystem properties. Variability, however, often depends on external factors that act as... | 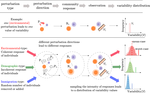 | Biodiversity, Coexistence, Community ecology, Competition, Interaction networks, Theoretical ecology | Kevin Cazelles | | 2018-10-02 14:01:03 | View |
Using a large-scale biodiversity monitoring dataset to test the effectiveness of protected areas at conserving North-American breeding birds
Victor Cazalis, Soumaya Belghali, Ana S.L. Rodrigues
https://doi.org/10.1101/433037
Protected Areas effects on biodiversity: a test using bird data that hopefully will give ideas for much more studies to come
Recommended by Paul Caplat based on reviews by Willson Gaul and 1 anonymous reviewer
In the face of worldwide declines in biodiversity, evaluating the effectiveness of conservation practices is an absolute necessity. Protected Areas (PA) are a key tool for conservation, and the question “Are PA effective” has been on many a research agenda, as the introduction to this preprint will no doubt convince you. A challenge we face is that, until now, few studies have been explicitly designed to evaluate PA, and despite the rise of meta-analyses on the topic, our capacity to quantify their effect on biodiversity remains limited.
This study by Cazalis et al. [1] uses the rich dataset of the North-American Breeding Bird Survey and a sound paired design to investigate how PA change bird assemblages. The methodological care brought to the study in itself is worth the read, and the results are insightful. I will not spoil too much by revealing here that things are “complicated”, and that effects – or lack thereof – depend on the type of ecosystem, and the type of species considered.
If you are interested in conservation, bird communities, species life-history, or like beautiful plots: go and read it.
References
[1] Cazalis, V., Belghali, S., & Rodrigues, A. S. (2019). Using a large-scale biodiversity monitoring dataset to test the effectiveness of protected areas at conserving North-American breeding birds. bioRxiv, 433037, ver. 4 peer-reviewed and recommended by PCI Ecology. doi: 10.1101/433037
| Using a large-scale biodiversity monitoring dataset to test the effectiveness of protected areas at conserving North-American breeding birds | Victor Cazalis, Soumaya Belghali, Ana S.L. Rodrigues | <p>Protected areas currently cover about 15% of the global land area, and constitute one of the main tools in biodiversity conservation. Quantifying their effectiveness at protecting species from local decline or extinction involves comparing prot... | 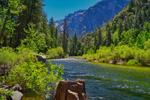 | Biodiversity, Conservation biology, Human impact, Landscape ecology, Macroecology | Paul Caplat | | 2018-10-04 08:43:34 | View |
Adapting to a changing environment: advancing our understanding of the mechanisms that lead to behavioral flexibility
Recommended by Erin Vogel based on reviews by Simon Gingins and 2 anonymous reviewers
Behavioral flexibility is essential for organisms to adapt to an ever-changing environment. However, the mechanisms that lead to behavioral flexibility and understanding what traits makes a species better able to adapt behavior to new environments has been understudied. Logan and colleagues have proposed to use a series of experiments, using great-tailed grackles as a study species, to test four main hypotheses. These hypotheses are centered around exploring the relationship between behavioral flexibility and inhibition in grackles. This current preregistration is a part of a larger integrative research plan examining behavioral flexibility when faced with environmental change. In this part of the project they will examine specifically if individuals that are more flexible are also better at inhibiting: in other words: they will test the assumption that inhibition is required for flexibility.
First, they will test the hypothesis that behavioral flexibility is manipulatable by using a serial reversal learning task. Second, they will test the hypothesis that manipulating behavioral flexibility (improving reversal learning speed through serial reversals using colored tubers) improves flexibility (rule switching) and problem solving in a new context (multi‑access box and serial reversals on a touch screen). Third, they will test the hypothesis that behavioral flexibility within a context is repeatable within individuals, which is important to test if performance is state dependent. Finally, they will test a fourth hypothesis that individuals should converge on an epsilon‑first learning strategy (learn the correct choice after one trial) as they progress through serial reversals. Their innovative approach using three main tasks (delay of gratification, go-no, detour) will allow them to assess different aspects of inhibitory control. They will analyze the results of all three experiments to also assess the utility of these experiments for studying the potential relationship between inhibition and behavioral flexibility.
In their preregistration, Logan and colleagues have proposed to test these hypotheses, each with a set of testable predictions that can be examined with detailed and justified methodologies. They have also provided a comprehensive plan for analyzing the data. All of the reviewers and I agree that this is a very interesting study that has the potential to answer important questions about a critical topic in behavioral ecology: the role of inhibition in the evolution of behavioral flexibility. Given the positive reviews, the comprehensive responses by the PI and her colleagues, and careful revisions, I highly recommend this preregistration.
| Are the more flexible great-tailed grackles also better at inhibition? | Corina Logan, Kelsey McCune, Zoe Johnson-Ulrich, Luisa Bergeron, Carolyn Rowney, Benjamin Seitz, Aaron Blaisdell, Claudia Wascher | This is a PREREGISTRATION. The DOI was issued by OSF and refers to the whole GitHub repository, which contains multiple files. The specific file we are submitting is g_inhibition.Rmd, which is easily accessible at GitHub at https://github.com/cori... | 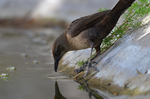 | Behaviour & Ethology, Preregistrations, Zoology | Erin Vogel | | 2018-10-12 18:36:00 | View |
Differential immune gene expression associated with contemporary range expansion of two invasive rodents in Senegal
Nathalie Charbonnel, Maxime Galan, Caroline Tatard, Anne Loiseau, Christophe Diagne, Ambroise Dalecky, Hugues Parrinello, Stephanie Rialle, Dany Severac and Carine Brouat
https://doi.org/10.1101/442160
Are all the roads leading to Rome?
Recommended by Simon Blanchet based on reviews by Nadia Aubin-Horth and 1 anonymous reviewer
Identifying the factors which favour the establishment and spread of non-native species in novel environments is one of the keys to predict - and hence prevent or control - biological invasions. This includes biological factors (i.e. factors associated with the invasive species themselves), and one of the prevailing hypotheses is that some species traits may explain their impressive success to establish and spread in novel environments [1]. In animals, most research studies have focused on traits associated with fecundity, age at maturity, level of affiliation to humans or dispersal ability for instance. The “composite picture” of the perfect (i.e. successful) invader that has gradually emerged is a small-bodied animal strongly affiliated to human activities with high fecundity, high dispersal ability and a super high level of plasticity. Of course, the story is not that simple, and actually a perfect invader sometimes – if not often- takes another form… Carrying on to identify what makes a species a successful invader or not is hence still an important research axis with major implications.
In this manuscript, Charbonnel and collaborators [2] provide an interesting opportunity to gain novel insights into our understanding of (the) traits underlying invasion success. They nicely combine the power of Next-Generation Sequencing (NGS) with a clever comparative approach of two closely-related invasive rodents (the house mouse Mus musculus and the black rat Rattus rattus) in a common environment. They use this experimental design to test the appealing hypothesis that pathogens may be actors of the story, and may indirectly explain why some non-native species are so successful in invading novel habitats.
It is generally assumed that the community of pathogens encountered by non-native species in novel environments is different from that of their native area. On the one hand (the enemy-release hypothesis), it can be hypothesized that non-native species, when they arrive into a novel environment, will be relaxed from the pressure imposed by their native pathogens because local pathogens are not adapted (and hence do not infect) to this novel host. Because immune defence against pathogens is highly costly, non-native species establishing into a novel environment could hence reallocate these costs to other functions such as fecundity or dispersal apparatus. This scenario has been termed the “evolution of increased competitive ability” (EICA) hypothesis [3]. On the other hand (the EICA-refined hypothesis [4]), one can assume that invaders will encounter new pathogens in newly established areas, and will allocate energy toward cost-effective immune pathways to permit allocating a non-negligible amount of energy toward other functions. Finally, a last hypothesis (the “immune protection” hypothesis) assumes major changes in pathogen composition between native and invaded areas, which should lead to an overall increase in immune investment by the native species to successfully invade novel environments [4]. This last hypothesis suggests that only non-native species being able to take up the associated costs of immunity will be successful invaders.
The role of immunity in invasion success has yet been poorly investigated, mainly because of the difficulty to simultaneously analyse multiple immune pathways [4]. Charbonnel and collaborators [2] overpass this difficulty by screening all genes expressed (using a whole RNA sequencing approach) in an immune tissue: the spleen. They do so along the invasion routes of two sympatric invasive rodents in Africa and compare anciently and newly invaded areas (respectively). For one of the two species (the house mouse), they found a high number of immune-related genes to be up-regulated in newly invaded areas compared to anciently invaded areas. All categories of immune pathways (costly and cost-effective) were up-regulated, suggesting an overall increase in immune investment in the mouse, which corroborates the “immune protection” hypothesis. For the black rat, patterns of gene expression were somewhat different, with much less pronounced differentiation in gene expression between newly and anciently invaded areas. Among the few differentiated genes, a few were associated to immune responses and some of theses genes were even down-regulated in the newly invaded areas. This pattern may actually corroborate the EICA hypothesis, although it could alternatively suggest that stochastic processes (drift) associated to recent decrease in population size (which is expected during a colonisation event) are more important than selection imposed by pathogens in shaping patterns of immune gene expression.
Overall, this study [2] suggests (i) that immune-related traits are important in predicting invasion success and (ii) that two successful species with a similar invasion history and living in similar environments can use different life-history strategies to reach the same success. This later finding is particularly relevant and intriguing as it suggests that the traits and strategies deployed by species to colonise new habitats might actually be idiosyncratic, and that, if general trends actually emerge in regards of traits predicting the success of invaders, the devil might actually be into the details. Comparative studies are extremely important to identify the general rules and the specificities sustaining actual patterns, but these approaches are yet poorly used in biological invasions (at least empirically). The work presented by Charbonnel and colleagues [2] calls for future comparative studies performed at multiple spatial scales (native vs. non-native areas, anciently vs. recently invaded areas), multiple taxonomic resolutions and across multiple traits (to search for trade-offs), so that the success of invasive species can be properly understood and predicted.
References
[1] Jeschke, J. M., & Strayer, D. L. (2006). Determinants of vertebrate invasion success in Europe and North America. Global Change Biology, 12(9), 1608-1619. doi: 10.1111/j.1365-2486.2006.01213.x
[2] Blossey, B., & Notzold, R. (1995). Evolution of increased competitive ability in invasive nonindigenous plants: a hypothesis. Journal of Ecology, 83(5), 887-889. doi: 10.2307/2261425
[3] Charbonnel, N., Galan, M., Tatard, C., Loiseau, A., Diagne, C. A., Dalecky, A., Parrinello, H., Rialle, S., Severac, D., & Brouat, C. (2019). Differential immune gene expression associated with contemporary range expansion of two invasive rodents in Senegal. bioRxiv, 442160, ver. 5 peer-reviewed and recommended by PCI Ecology. doi: 10.1101/442160
[4] Lee, K. A., & Klasing, K. C. (2004). A role for immunology in invasion biology. Trends in Ecology & Evolution, 19(10), 523-529. doi: 10.1016/j.tree.2004.07.012
| Differential immune gene expression associated with contemporary range expansion of two invasive rodents in Senegal | Nathalie Charbonnel, Maxime Galan, Caroline Tatard, Anne Loiseau, Christophe Diagne, Ambroise Dalecky, Hugues Parrinello, Stephanie Rialle, Dany Severac and Carine Brouat | <p>Background: Biological invasions are major anthropogenic changes associated with threats to biodiversity and health. What determines the successful establishment of introduced populations still remains unsolved. Here we explore the appealing as... | 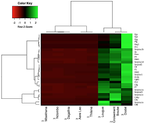 | Biological invasions, Eco-immunology & Immunity, Population ecology | Simon Blanchet | | 2018-10-14 12:21:52 | View |
Direct and transgenerational effects of an experimental heat wave on early life stages in a freshwater snail
Katja Leicht, Otto Seppälä
https://doi.org/10.1101/449777
Escargots cooked just right: telling apart the direct and indirect effects of heat waves in freashwater snails
Recommended by vincent calcagno based on reviews by Amanda Lynn Caskenette, Kévin Tougeron and arnaud sentis
Amongst the many challenges and forms of environmental change that organisms face in our era of global change, climate change is perhaps one of the most straightforward and amenable to investigation. First, measurements of day-to-day temperatures are relatively feasible and accessible, and predictions regarding the expected trends in Earth surface temperature are probably some of the most reliable we have. It appears quite clear, in particular, that beyond the overall increase in average temperature, the heat waves locally experienced by organisms in their natural habitats are bound to become more frequent, more intense, and more long-lasting [1]. Second, it is well appreciated that temperature is a major environmental factor with strong impacts on different facets of organismal development and life-history [2-4]. These impacts have reasonably clear mechanistic underpinnings, with definite connections to biochemistry, physiology, and considerations on energetics. Third, since variation in temperature is a challenge already experienced by natural populations across their current and historical ranges, it is not a completely alien form of environmental change. Therefore, we already learnt quite a lot about it in several species, and so did the species, as they may be expected to have evolved dedicated adaptive mechanisms to respond to elevated temperatures. Last, but not least, temperature is quite amenable to being manipulated as an experimental factor.
For all these reasons, experimental studies of the consequences of increased temperature hit some of a sweetspot and are a source of very nice research, in many different organisms. The work by Leicht and Seppala [5] complements a sequence of earlier studies by this group, using the freshwater snail Lymnaea stagnalis as their model system [6-7].
In the present study, the authors investigate how a heat wave (a period of abnormally elevated temperature, here 25°C versus a normal 15°C) may have indirect effects on the next generation, through maternal effects. They question whether such indirect effects exist, and if they exist, how they compare, in terms of effect size, with the (more straightforward) direct effects observed in individuals that directly experience a heat wave. Transgenerational effects are well-known to occur following periods of physiological stress, and might thus have non negligible contributions to the overall effect of warming.
In this freshwater snail, heat has very strong direct effects: mortality increases at high temperature, but survivors grow much bigger, with a greater propensity to lay eggs and a (spectacular) three-fold increase in the number of eggs laid [6]. Considering that, it is easy to consider that transgenerational effects should be small game. And indeed, the present study also observes the big and obvious direct effects of elevated temperature: higher mortality, but greater propensity to oviposit. However, it was also found that the eggs were smaller if from mothers exposed to high temperature, with a correspondingly smaller size of hatchlings. This suggests that a heat wave causes the snails to lay more eggs, but smaller ones, reminiscent of a size-number trade-off. Unfortunately, clutch size could not be measured in this experiment, so this cannot be investigated any further. For this trait, the indirect effect may indeed be regarded as small game : eggs and hatchlings were about 15 % smaller, an effect size pretty small compared to the mammoth direct positive effect of temperature on shell length (see Figure 4 ; and also [6]). The same is true for developmental time (Figure 3).
However, for some traits the story was different. In particular, it was found that the (smaller) eggs produced from heated mothers were more likely to hatch by almost 10% (Figure 2). Here the indirect effect not only goes against the direct effect (hatching rate is lower at high temperature), but it also has similar effect size. As a consequence, taking into account both the indirect and direct effects, hatching success is essentially the same at 15°C and 25°C (Figure 2). Survival also had comparable effect sizes for direct and indirect effects. Indeed, survival was reduced by about 20% regardless of whom endured the heat stress (the focal individual or her mother; Figure 4). Interestingly, the direct and indirect effects were not quite cumulative: if a mother experienced a heat wave, heating up the offspring did not do much more damage, as though the offspring were ‘adapted’ to the warmer conditions (but keep in mind that, surprisingly, the authors’ stats did not find a significant interaction; Table 2).
At the end of the day, even though at first heat seems a relatively simple and understandable component of environmental change, this study shows how varied its effects can be effects on different components of individual fitness. The overall impact most likely is a mix of direct and indirect effects, of shifts along allocation trade-offs, and of maladaptive and adaptive responses, whose overall ecological significance is not so easy to grasp. That said, this study shows that direct and indirect (maternal) effects can sometimes go against one another and have similar intensities. Indirect effects should therefore not be overlooked in this kind of studies. It also gives a hint of what an interesting challenge it is to understand the adaptive or maladaptive nature of organism responses to elevated temperatures, and to evaluate their ultimate fitness consequences.
References
[1] Meehl, G. A., & Tebaldi, C. (2004). More intense, more frequent, and longer lasting heat waves in the 21st century. Science (New York, N.Y.), 305(5686), 994–997. doi: 10.1126/science.1098704
[2] Adamo, S. A., & Lovett, M. M. E. (2011). Some like it hot: the effects of climate change on reproduction, immune function and disease resistance in the cricket Gryllus texensis. The Journal of Experimental Biology, 214(Pt 12), 1997–2004. doi: 10.1242/jeb.056531
[3] Deutsch, C. A., Tewksbury, J. J., Tigchelaar, M., Battisti, D. S., Merrill, S. C., Huey, R. B., & Naylor, R. L. (2018). Increase in crop losses to insect pests in a warming climate. Science (New York, N.Y.), 361(6405), 916–919. doi: 10.1126/science.aat3466
[4] Sentis, A., Hemptinne, J.-L., & Brodeur, J. (2013). Effects of simulated heat waves on an experimental plant–herbivore–predator food chain. Global Change Biology, 19(3), 833–842. doi: 10.1111/gcb.12094
[5] Leicht, K., & Seppälä, O. (2019). Direct and transgenerational effects of an experimental heat wave on early life stages in a freshwater snail. BioRxiv, 449777, ver. 4 peer-reviewed and recommended by PCI Ecology. doi: 10.1101/449777
[6] Leicht, K., Seppälä, K., & Seppälä, O. (2017). Potential for adaptation to climate change: family-level variation in fitness-related traits and their responses to heat waves in a snail population. BMC Evolutionary Biology, 17(1), 140. doi: 10.1186/s12862-017-0988-x
[7] Leicht, K., Jokela, J., & Seppälä, O. (2013). An experimental heat wave changes immune defense and life history traits in a freshwater snail. Ecology and Evolution, 3(15), 4861–4871. doi: 10.1002/ece3.874
| Direct and transgenerational effects of an experimental heat wave on early life stages in a freshwater snail | Katja Leicht, Otto Seppälä | <p>Global climate change imposes a serious threat to natural populations of many species. Estimates of the effects of climate change‐mediated environmental stresses are, however, often based only on their direct effects on organisms, and neglect t... | 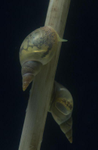 | Climate change | vincent calcagno | | 2018-10-22 22:19:22 | View |
Understanding geographic range expansions in human-dominated landscapes: does behavioral flexibility modulate flexibility in foraging and social behavior?
Recommended by Julia Astegiano and Esther Sebastián González based on reviews by Pizza Ka Yee Chow and Esther Sebastián González
Which biological traits modulate species distribution has historically been and still is one of the core questions of the macroecology and biogeography agenda [1, 2]. As most of the Earth surface has been modified by human activities [3] understanding the strategies that allow species to inhabit human-dominated landscapes will be key to explain species geographic distribution in the Anthropocene. In this vein, Logan et al. [4] are working on a long-term and integrative project aimed to investigate how great-tailed grackles rapidly expanded their geographic range into North America [4]. Particularly, they want to determine which is the role of behavioral flexibility, i.e. an individual’s ability to modify its behavior when circumstances change based on learning from previous experience [5], in rapid geographic range expansions. The authors are already working in a set of complementary questions described in pre-registrations that have already been recommended at PCI Ecology: (1) Do individuals with greater behavioral flexibility rely more on causal cognition [6]? (2) Which are the mechanisms that lead to behavioral flexibility [7]? (3) Does the manipulation of behavioral flexibility affect exploration, but not boldness, persistence, or motor diversity [8]? (4) Can context changes improve behavioral flexibility [9]?
In this new pre-registration, they aim to determine whether the more behaviorally flexible individuals have more flexible foraging behaviors (i.e. use a wider variety of foraging techniques in the wild and eat a larger number of different foods), habitat use (i.e. higher microhabitat richness) and social relationships (i.e., are more likely to have a greater number of bonds or stronger bonds with other individuals; [4]). The project is ambitious, combining both the experimental characterization of individuals’ behavioral flexibility and the field characterization of the foraging and social behavior of those individuals and of wild ones.
The current great-tailed grackles project will be highly relevant to understand rapid geographic range expansions in a changing world. In this vein, this pre-registration will particularly help to go one step further in our understanding of behavioral flexibility as a determinant of species geographic distribution. Logan et al. [4] pre-registration is very well designed, main and alternative hypotheses have been thought and written and methods are presented in a very detailed way, which includes the R codes that authors will use in their analyses. Authors have answered in a very detailed way each comment that reviewers have pointed out and modified the pre-registration accordingly, which we consider highly improved the quality of this work. That is why we strongly recommend this pre-registration and look forward to see the results.
References
[1] Gaston K. J. (2003) The structure and dynamics of geographic ranges. Oxford series in Ecology and Evolution. Oxford University Press, New York.
[2] Castro-Insua, A., Gómez‐Rodríguez, C., Svenning, J.C., and Baselga, A. (2018) A new macroecological pattern: The latitudinal gradient in species range shape. Global ecology and biogeography, 27(3), 357-367. doi: 10.1111/geb.12702
[3] Newbold, T., Hudson, L. N., Hill, S. L. L., Contu, S., Lysenko, I., Senior, R. A., et al. (2015). Global effects of land use on local terrestrial biodiversity. Nature, 520(7545), 45–50. doi: 10.1038/nature14324
[4] Logan CJ, McCune K, Bergeron L, Folsom M, Lukas D. (2019). Is behavioral flexibility related to foraging and social behavior in a rapidly expanding species? In principle recommendation by Peer Community In Ecology. http://corinalogan.com/Preregistrations/g_flexforaging.html
[5] Mikhalevich, I., Powell, R., and Logan, C. (2017). Is Behavioural Flexibility Evidence of Cognitive Complexity? How Evolution Can Inform Comparative Cognition. Interface Focus 7: 20160121. doi: 10.1098/rsfs.2016.0121.
[6] Fronhofer, E. (2019) From cognition to range dynamics: advancing our understanding of macroecological patterns. Peer Community in Ecology, 100014. doi: 10.24072/pci.ecology.100014
[7] Vogel, E. (2019) Adapting to a changing environment: advancing our understanding of the mechanisms that lead to behavioral flexibility. Peer Community in Ecology, 100016. doi: 10.24072/pci.ecology.100016
[8] Van Cleve, J. (2019) Probing behaviors correlated with behavioral flexibility. Peer Community in Ecology, 100020. doi: 10.24072/pci.ecology.100020
[9] Coulon, A. (2019) Can context changes improve behavioral flexibility? Towards a better understanding of species adaptability to environmental changes. Peer Community in Ecology, 100019. doi: 10.24072/pci.ecology.100019
| Is behavioral flexibility related to foraging and social behavior in a rapidly expanding species? | Corina Logan, Luisa Bergeron, Carolyn Rowney, Kelsey McCune, Dieter Lukas | This is one of the first studies planned for our long-term research on the role of behavioral flexibility in rapid geographic range expansions. Project background: Behavioral flexibility, the ability to change behavior when circumstances change ba... | 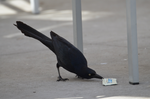 | Behaviour & Ethology, Preregistrations, Zoology | Julia Astegiano | | 2018-10-23 00:47:03 | View |
Interplay between the paradox of enrichment and nutrient cycling in food webs
Pierre Quévreux, Sébastien Barot and Élisa Thébault
https://doi.org/10.1101/276592
New insights into the role of nutrient cycling in food web dynamics
Recommended by Samraat Pawar based on reviews by Jean-François Arnoldi, Wojciech Uszko and 1 anonymous reviewer
Understanding the factors that govern the relationship between structure, stability and functioning of food webs has been a central problem in ecology for many decades. Historically, apart from microbial and soil food webs, the role of nutrient cycling has largely been ignored in theoretical and empirical food web studies. A prime example of this is the widespread use of Lotka-Volterra type models in theoretical studies; these models per se are not designed to capture the effect of nutrients being released back into the system by interacting populations. Thus overall, we still lack a general understanding of how nutrient cycling affects food web dynamics.
A new study by Quévreux, Barot and Thébault [1] tackles this problem by building a new food web model. This model features some important biological details: trophic interactions and vital rates constrained by species' body masses (using Ecological Metabolic Theory), adaptive foraging, and stoichiometric rules to ensure meaningful conversion between carbon and nutrient flows. The authors analyze the model through detailed simulations combined with thorough sensitivity analyses of model assumptions and parametrizations (including of allometric scaling relationships). I am happy to recommend this preprint because of the novelty of the work and it's technical quality.
The study yields interesting and novel findings. Overall, nutrient cycling does have a strong effect on community dynamics. Nutrient recycling is driven mostly by consumers at low mineral nutrient inputs, and by primary producers at high inputs. The extra nutrients made available through recycling increases species' persistence at low nutrient input levels, but decreases persistence at higher input levels by increasing population oscillations (a new, nuanced perspective on the classical "paradox of enrichment"). Also, for the same level of nutrient input, food webs with nutrient recycling show more fluctuations in primary producer biomass (and less at higher trophic levels) than those without recycling, with this effect weakening in more complex food webs.
Overall, these results provide new insights, suggesting that nutrient cycling may enhance the positive effects of species richness on ecosystem stability, and point at interesting new directions for future theoretical and empirical studies.
References
[1] Quévreux, P., Barot, S. and E. Thébault (2020) Interplay between the paradox of enrichment and nutrient cycling in food webs. bioRxiv, 276592, ver. 7 peer-reviewed and recommended by PCI Ecology. doi: 10.1101/276592
| Interplay between the paradox of enrichment and nutrient cycling in food webs | Pierre Quévreux, Sébastien Barot and Élisa Thébault | <p>Nutrient cycling is fundamental to ecosystem functioning. Despite recent major advances in the understanding of complex food web dynamics, food web models have so far generally ignored nutrient cycling. However, nutrient cycling is expected to ... | 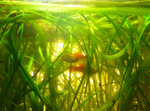 | Biodiversity, Community ecology, Ecosystem functioning, Food webs, Interaction networks, Theoretical ecology | Samraat Pawar | | 2018-11-03 21:47:37 | View |
Evaluating functional dispersal and its eco-epidemiological implications in a nest ectoparasite
Amalia Rataud, Marlène Dupraz, Céline Toty, Thomas Blanchon, Marion Vittecoq, Rémi Choquet, Karen D. McCoy
https://doi.org/10.5281/zenodo.2592114
Limited dispersal in a vector on territorial hosts
Recommended by Adele Mennerat based on reviews by Shelly Lachish and 1 anonymous reviewer
Parasitism requires parasites and hosts to meet and is therefore conditioned by their respective dispersal abilities. While dispersal has been studied in a number of wild vertebrates (including in relation to infection risk), we still have poor knowledge of the movements of their parasites. Yet we know that many parasites, and in particular vectors transmitting pathogens from host to host, possess the ability to move actively during at least part of their lives.
So... how far does a vector go – and is this reflected in the population structure of the pathogens they transmit? This is the question addressed by Rataud et al. [1], who provide the first attempt at using capture-mark-recapture to estimate not only functional dispersal, but also detection probability and survival in a wild parasite that is also a vector for other pathogens.
The authors find that (i) functional dispersal of soft ticks within a gull colony is very limited. Moreover, they observe unexpected patterns: (ii) experimental displacement of ticks does not induce homing behaviour, and (iii) despite lower survival, tick dispersal was lower in nests not containing hosts than in successful nests.
These results contrast with expectations based on the distribution of infectious agents. Low tick dispersal within the colony, combined with host territoriality during breeding and high site fidelity between years should result in a spatially structured distribution of infectious agents carried by ticks. This is not the case here. One possible explanation could be that soft ticks live for much longer than a breeding season, and that they disperse at other times of year to a larger extent than usually assumed.
This study represents one chapter of a story that will likely keep unfolding. It raises fascinating questions, and illustrates the importance of basic knowledge of parasite ecology and behaviour to better understand pathogen dynamics in the wild.
References
[1] Rataud A., Dupraz M., Toty C., Blanchon T., Vittecoq M., Choquet R. & McCoy K.D. (2019). Evaluating functional dispersal and its eco-epidemiological implications in a nest ectoparasite. Zenodo, 2592114. Ver. 3 peer-reviewed and recommended by PCI Ecology. doi: 10.5281/zenodo.2592114
| Evaluating functional dispersal and its eco-epidemiological implications in a nest ectoparasite | Amalia Rataud, Marlène Dupraz, Céline Toty, Thomas Blanchon, Marion Vittecoq, Rémi Choquet, Karen D. McCoy | <p>Functional dispersal (between-site movement, with or without subsequent reproduction) is a key trait acting on the ecological and evolutionary trajectories of a species, with potential cascading effects on other members of the local community. ... | 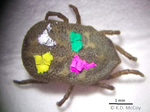 | Dispersal & Migration, Epidemiology, Parasitology, Population ecology | Adele Mennerat | | 2018-11-05 11:44:58 | View |