
RÉALE Denis
- Département des sciences biologiques, UQAM, Montreal, Canada
- Behaviour & Ethology, Eco-evolutionary dynamics, Evolutionary ecology, Life history, Maternal effects, Phenotypic plasticity, Population ecology
- recommender
Recommendations: 3
Reviews: 0
Recommendations: 3
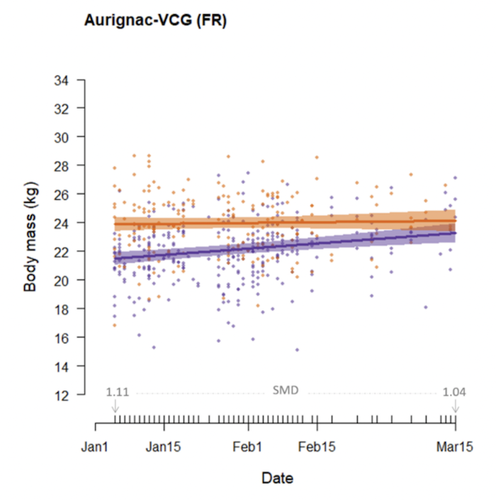
Body mass change over winter is consistently sex-specific across roe deer (Capreolus capreolus) populations
Is it sexual mass dimorphism season?
Recommended by Denis Réale based on reviews by Patrick Bergeron, Philip McLoughlin and Achaz von HardenbergPolygyny is assumed to have led to the evolution of strong sexual size dimorphism (SSD) in mammals, males often being heavier or showing more developed armaments than females (Weckerly 1998; Loison et al. 1999; Pérez‐Barbería et al. 2002). SSD generally increases with the degree of polygyny of the species. However, the degree of SSD, and particularly of sexual mass dimorphism, is not fixed for each species, and differences exist between populations (Blanckenhorn et al. 2006; Cox & Calsbeek 2010) or even between seasons within populations (Rughetti & Festa‐Bianchet 2011).
In this study, Hewison et al. propose that studying seasonal variation in sexual mass dimorphism and how this can be affected by winter harshness and latitude allows us to better assess the energetic costs associated with the eco-evolutionary constraints acting on each sex. To achieve their goal, Hewison et al. use a formidable, long-term dataset of over 7,000 individuals, in five roe deer populations (Capreolus capreolus), from south-west France and Sweden.
According to the authors, sexual mass dimorphism should be at its lowest in early spring in this species due to a stronger trade-off between antler growth and body weight maintenance in males over winter than in females. Furthermore, harsher conditions, varying both in time and space (i.e., Sweden vs. France), should increase winter weight loss, and thus, mass change differences between the sexes should be stronger and show more variation in Sweden than in France.
Their results support their hypotheses. In the two Swedish populations, males lost more mass than females. In the three French populations, males maintain their body mass while females gain some over the winter. Because of these sex-dependent loss/gain in body mass, sexual dimorphism was stronger early in the winter and null at the onset of spring. Furthermore, sexual dimorphism was stronger in southern than in northern populations. In France, males weighed about 10% more than females, while they weighed about 5% more in Sweden. Roe deer, however, do not show any dimorphism early in the spring, when males start defending their territory.
The authors also found more variation in mass change among years in Swedish than in French roe deer, suggesting a stronger effect of winter severity on the dynamics of mass change in northern than in southern populations. The authors interpret the decrease in sexual dimorphism throughout the winter by the fact that, during this period, the energetic cost paid by males associated with the growth of their antlers and the effort of establishing their mating territory. They thus attribute the greater mass change in males to the competitive allocation of resources to antler growth or body mass. They also discuss the low probability that such sex differences in mass change could be caused by females’ gestation in this species.
Interestingly, Hewison et al. found that individual differences represented more than 70% of the total variation in body mass, and the low estimated among-individual variance in slopes with time might indicate that, despite a lower SSD, selection pressures on body mass can still be maintained at times when body mass may play an important role, such as in spring with territorial defense or later during mating (Vanpé et al. 2010).
I recommend this article because it produces strong results, which show, without a shadow of a doubt, sex differences in their seasonal mass changes, resulting in a marked seasonal variation in SSD. The differences observed between southern and northern populations confirm the idea that the severity of the winters endured by these populations acts as a constraint on the deer's patterns of mass change. I hope this study will encourage more examinations of how eco-evolutionary constraints affect the sexual size dimorphism.
References
Blanckenhorn, W. U., Stillwell, R. C., Young, K. A., Fox, C. W., & Ashton, K. G. (2006). When Rensch meets Bergmann: does sexual size dimorphism change systematically with latitude? Evolution, 60(10), 2004-2011. https://doi.org/10.1554/06-110.1
Cox, R. M., & Calsbeek, R. (2010). Sex-specific selection and intraspecific variation in sexual size dimorphism. Evolution, 64(3), 798-809. https://doi.org/10.1111/j.1558-5646.2009.00851.x
Hewison M, Bonnot N, Gaillard JM, Kjellander P, Lemaitre J-F, Morellet N. and Pellerin M (2024) Body mass change over winter is consistently sex-specific across roe deer (Capreolus capreolus) populations. bioRxiv, ver.4 peer-reviewed and recommended by PCI Ecology https://doi.org/10.1101/2022.09.09.507329
Loison, A., Gaillard, J. M., Pélabon, C., & Yoccoz, N. G. (1999). What factors shape sexual size dimorphism in ungulates? Evolutionary Ecology Research, 1(5), 611-633. https://www.evolutionary-ecology.com/issues/v01n05/jjar1019.pdf
Pérez‐Barbería, F. J., Gordon, I. J., & Pagel, M. (2002). The origins of sexual dimorphism in body size in ungulates. Evolution, 56(6), 1276-1285. https://doi.org/10.1111/j.0014-3820.2002.tb01438.x
Rughetti, M., & Festa‐Bianchet, M. (2011). Seasonal changes in sexual size dimorphism in northern chamois. Journal of Zoology, 284(4), 257-264. https://doi.org/10.1111/j.1469-7998.2011.00800.x
Vanpé, C., Gaillard, J. M., Kjellander, P., Liberg, O., Delorme, D., & Hewison, A. M. (2010). Assessing the intensity of sexual selection on male body mass and antler length in roe deer Capreolus capreolus: is bigger better in a weakly dimorphic species? Oikos, 119(9), 1484-1492. https://doi.org/10.1111/j.1600-0706.2010.18312.x
Weckerly, F. W. (1998). Sexual-size dimorphism: influence of mass and mating systems in the most dimorphic mammals. Journal of Mammalogy, 79(1), 33-52. https://doi.org/10.2307/1382840
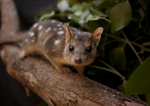
Trophic cascade driven by behavioural fine-tuning as naïve prey rapidly adjust to a novel predator
While the quoll’s away, the mice will play… and the seeds will pay
Recommended by Denis Réale based on reviews by 2 anonymous reviewersA predator can strongly influence the demography of its prey, which can have profound carryover effects on the trophic network; so-called density-mediated indirect interactions (DMII; Werner and Peacor 2003; Schmitz et al. 2004; Trussell et al. 2006). Furthermore, a novel predator can alter the phenotypes of its prey for traits that will change prey foraging efficiency. These trait-mediated indirect interactions may in turn have cascading effects on the demography and features of the basal resources consumed by the intermediate consumer (TMIII; Werner and Peacor 2003; Schmitz et al. 2004; Trussell et al. 2006), but very few studies have looked for these effects (Trusell et al. 2006). The study “Trophic cascade driven by behavioural fine-tuning as naïve prey rapidly adjust to a novel predator”, by Jolly et al. (2020) is therefore a much-needed addition to knowledge in this field. The authors have profited from a rare introduction of Northern quolls (Dasyurus hallucatus) on an Australian island, to examine both the density-mediated and trait-mediated indirect interactions with grassland melomys (Melomys burtoni) and the vegetation of their woodland habitat.
Jolly et al. (2020) compared melomys populations in four quoll-invaded and three quoll-free sites on the same island. Using capture-mark-recapture methods, they found a lower survival and decreased population size in quoll-invaded sites compared to quoll-free sites. Although they acknowledge that this decline could be attributable to either the direct effects of the predator or to a wildfire that occurred early in the experiment in the quoll-invaded sites, the authors argue that the wildfire alone cannot explain all of their results.
Beyond demographic effects, Jolly et al. (2020) also examined risk taking, foraging behaviour, and predator avoidance in melomys. Quoll presence was first associated with a strong decrease in risk taking in melomys, but the difference disappeared over the three years of study, indicating a possible adjustment by the prey. In quoll-invaded sites, though, melomys continued to be more neophobic than in the quoll-free sites throughout the study. Furthermore, in a seed (i.e. wheat) removal experiment, Jolly et al. (2020) measured how melomys harvested seeds in the presence or absence of predator scents. In both quoll-invaded and quoll-free sites, melomys density increased seed harvest efficiency. Melomys also removed less seeds in quoll-invaded sites than in quoll-free sites, supporting both the DMII and TMII hypotheses. However, in the quoll-invaded sites only, melomys foraged less on predator-scented seed patches than on unscented ones, trading foraging efficiency for an increased safety against predators, and this effect increased across the years. This last result indicates that predators can indirectly influence seed consumption through the trade-off between foraging and predator avoidance, strongly supporting the TMII hypothesis.
Ideally, the authors would have run a nice before-after, impact-control design, but nature does not always allow for ideal experimental designs. Regardless, the results of such an “experiment in the wild” predation study are still valuable, as they are very rare (Trussell et al. 2006), and they provide crucial information on the direct and indirect interactions along a trophic cascade. Furthermore, the authors have effectively addressed any concerns about potential confounding factors, and thus have a convincing argument that their results represent predator-driven demographic and behavioural changes.
One important question remains from an evolutionary ecology standpoint: do the responses of melomys to the presence of quolls represent phenotypically plastic changes or rapid evolutionary changes caused by novel selection pressures? Classically, TMII are assumed to be mostly caused by phenotypic plasticity (Werner and Peacor 2003), and this might be the case when the presence of the predator is historical. Phenotypic plasticity allows quick and reversible adjustments of the prey population to changes in the predator density. When the predator population declines, such rapid phenotypic changes can be reversed, reducing the cost associated with anti-predator behaviour (e.g., lower foraging efficiency) in the absence of predators. In the case of a novel predator, however, short-term evolutionary responses by the prey may play role in the TMII, as they would allow a phenotypic shift in prey’s traits along the trade-off between foraging efficiency and anti-predator response that will probably more advantageous over the longer term, if the predator does not disappear. The authors state that they could not rule out one or the other of these hypotheses. However, future work estimating the relative importance of phenotypic plasticity and evolutionary changes in the quoll-melomys system would be valuable. Phenotypic selection analysis, for example, by estimating the link between survival and the traits measured, might help test for a fitness advantage to altered behaviour in the presence of a predator. Common garden experiments, comparing the quoll-invaded and the quoll-free melomys populations, might also provide information on any potential evolutionary changes caused by predation. More work could also analyse the potential effects on the seed populations. Not only might the reduction in seed predation have consequences on the landscape in the future, as the authors mention, but it may also mean that the seeds themselves could be subject to novel selection pressures, which may affect their phenology, physiology or life history. Off course, the authors will have to switch from wheat to a more natural situation, and evaluate the effects of changes in the melomys population on the feature of the local vegetation and the ecosystem.
Finally, the authors have not yet found that the observed changes in the traits have translated into a demographic rebound for melomys. Here again, I can see an interesting potential for further studies. Should we really expect an evolutionary rescue (Bell and Gonzalez 2009) in this system? Alternatively, should the changes in behaviour be accompanied by permanent changes in life history, such as a slower pace-of-life (Réale et al. 2010) that could possibly lead to lower melomys density?
This paper provides nice in natura evidence for density- and trait-mediated indirect interactions hypotheses. I hope it will be the first of a long series of work on this interesting quoll-melomys system, and that the authors will be able to provide more information on the eco-evolutionary consequences of a novel predator on a trophic network.
References
-Bell G, Gonzalez A (2009) Evolutionary rescue can prevent extinction following environmental change. Ecology letters, 12(9), 942-948. https://doi.org/10.1111/j.1461-0248.2009.01350.x
-Jolly CJ, Smart AS, Moreen J, Webb JK, Gillespie GR, Phillips BL (2020) Trophic cascade driven by behavioural fine-tuning as naïve prey rapidly adjust to a novel predator. bioRxiv, 856997, ver. 6 peer-reviewed and recommended by PCI Ecology. https://doi.org/ 10.1101/856997
-Matassa C, Ewanchuk P, Trussell G (2018) Cascading effects of a top predator on intraspecific competition at intermediate and basal trophic levels. Functional Ecology, 32(9), 2241-2252. https://doi.org/10.1111/1365-2435.13131
-Réale D, Garant D, Humphries MM, Bergeron P, Careau V, Montiglio PO (2010) Personality and the emergence of the pace-of-life syndrome concept at the population level. Philosophical Transactions of the Royal Society B: Biological Sciences, 365(1560), 4051-4063. https://doi.org/10.1098/rstb.2010.0208
-Schmitz O, Krivan V, Ovadia O (2004) Trophic cascades: the primacy of trait‐mediated indirect interactions. Ecology Letters 7(2), 153-163. https://doi.org/10.1111/j.1461-0248.2003.00560.x
-Trussell G, Ewanchuk P, Matassa C (2006). Habitat effects on the relative importance of trait‐ and density‐mediated indirect interactions. Ecology Letters, 9(11), 1245-1252. https://doi.org/10.1111/j.1461-0248.2006.00981.x
-Werner EE, Peacor SD (2003) A review of trait‐mediated indirect interactions in ecological communities. Ecology, 84(5), 1083-1100. https://doi.org/10.1890/0012-9658(2003)084[1083:AROTII]2.0.CO;2
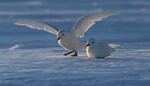
Sexual segregation in a highly pagophilic and sexually dimorphic marine predator
Sexual segregation in a sexually dimorphic seabird: a matter of spatial scale
Recommended by Denis Réale based on reviews by Dries Bonte and 1 anonymous reviewerSexual segregation appears in many taxa and can have important ecological, evolutionary and conservation implications. Sexual segregation can take two forms: either the two sexes specialise in different habitats but share the same area (habitat segregation), or they occupy the same habitat but form separate, unisex groups (social segregation) [1,2]. Segregation would have evolved as a way to avoid, or at least, reduce intersexual competition.
Testing whether social or habitat segregation is at play necessitates the use of combined approaches to determine the spatial scale at which segregation occurs. This enterprise is even more challenging when studying marine species, which travel over long distances to reach their foraging areas. This is what Barbraud et al. [3] have endeavoured on the snow petrel (Pagodroma nivea), a sexually dimorphic, polar seabird. Studying sexual segregation at sea requires tools for indirect measures of habitat use and foraging tactics. During the incubation period, in a colony based at Pointe Geologie, Adelie land, East Antarctica, the team has equipped birds with GPS loggers to analyse habitat use and foraging behaviour. It has also compared short-, mid-, and long-term stable isotopic profiles, from plasma, blood cells, and feather samples, respectively.
Barbraud et al. [3] could not detect any evidence for sexual segregation in space use. Furthermore, the two sexes showed similar δ13C profiles, illustrating similar foraging latitudes, and indicating no sexual segregation at large spatial scales. Snow petrels all forage exclusively in the sea ice environment formed over the deep Antarctic continental shelf. The authors, however, found other forms of segregation: males consistently foraged at higher sea ice concentrations than females. Males also fed on higher trophic levels than females. Therefore, male and female snow petrels segregate at a smaller spatial scale, and use different foraging tactics and diet specialisations. Females also took shorter foraging trips than males, with higher mass gain that strongly benefit from higher sea ice concentration. Mass gain in males increased with the length of their foraging trip at sea ice areas.
The authors conclude that high sea ice concentration offers the most favourable foraging habitat for snow petrels, and thus that intersexual competition may drive females away from high sea ice areas. This study shows that combining information from different tools provides an elegant way of isolating the potential factors driving sexual segregation and the spatial scales at which it occurs.
References
[1] Conradt, L. (2005). Definitions, hypotheses, models and measures in the study of animal segregation. In Sexual segregation in vertebrates: ecology of the two sexes (Ruckstuhl K.E. and Neuhaus, P. eds). Cambridge University Press, Cambridge, United Kingdom. Pp:11–34.
[2] Ruckstuhl, K. E. (2007). Sexual segregation in vertebrates: proximate and ultimate causes. Integrative and Comparative Biology, 47(2), 245-257. doi: 10.1093/icb/icm030
[3] Barbraud, C., Delord, K., Kato, A., Bustamante, P., & Cherel, Y. (2018). Sexual segregation in a highly pagophilic and sexually dimorphic marine predator. bioRxiv, 472431, ver. 3 peer-reviewed and recommended bt PCI Ecology. doi: 10.1101/472431